type
status
date
slug
summary
tags
category
icon
password
High Cell Selectivity and Bactericidal Mechanism of Symmetric Peptides Centered on d-Pro–Gly Pairs以 d-Pro-Gly 对为中心的对称肽的高细胞选择性和杀菌机制
by Boyan Jia 1,†,Yiming Wang 1,†,Ying Zhang 1,Zi Wang 2,Xue Wang 1,Inam Muhammad 1,Lingcong Kong 1,Zhihua Pei 1,Hongxia Ma 1,* andXiuyun Jiang 1,3,4,*
1
College of Animal Science and Technology, Jilin Agricultural University, Xincheng Street No.#2888, Changchun 130118, China吉林农业大学动物科技学院, 长春市新城大街2888号 130118
2
College of Animal Science and Technology, Inner Mongolia University for Nationalities, Tongliao 028000, China内蒙古民族大学动物科技学院, 通辽 028000
3
College of Life Science, Jilin Agricultural University, Xincheng Street No.#2888, Changchun 130118, China吉林农业大学生命科学学院, 长春市新城大街2888号 130118
4
College of Animal Science and Technology, Changchun Sci-Tech University, Changchun 130600, China长春理工大学动物科技学院, 长春 130600
Authors to whom correspondence should be addressed.应向其通信的作者。
†
These authors contributed equally to this work.这些作者同等贡献这项工作。
Int. J. Mol. Sci. 2020, 21(3), 1140; https://doi.org/10.3390/ijms21031140
Submission received: 1 January 2020 / Revised: 2 February 2020 / Accepted: 4 February 2020 / Published: 8 February 2020收到提交材料:2020 年 1 月 1 日 / 修订:2020 年 2 月 2 日 / 接受:2020 年 2 月 4 日 / 发布:2020 年 2 月 8 日
(This article belongs to the Special Issue Biochemistry, Molecular Biology and Druggability of Proteins)(本文属于特刊《生物化学、分子生物学和蛋白质的成药性》)
Downloadkeyboard_arrow_down
AbstractAntimicrobial peptides (AMPs) have a unique action mechanism that can help to solve global problems in antibiotic resistance. However, their low therapeutic index and poor stability seriously hamper their development as therapeutic agents. 摘要 抗菌肽(AMPs)具有独特的作用机制,有助于解决全球性的抗生素耐药性问题。然而,它们的低治疗指数和差的稳定性严重阻碍了它们作为治疗剂的发展。In order to overcome these problems, we designed peptides based on the sequence template XXRXXRRzzRRXXRXX-NH2, where X represents a hydrophobic amino acid like Phe (F), Ile (I), and Leu (L), while zz represents Gly–Gly (GG) or d-Pro–Gly (pG). 为了克服这些问题,我们基于序列模板XXRXXRRzzRRXXRXX-NH2设计了肽,其中X代表疏水性氨基酸,如Phe(F)、Ile(I)和Leu(L),而zz代表Gly–Gly( GG) 或 d-Pro-Gly (pG)。Showing effective antimicrobial activity against Gram-negative bacteria and low toxicity, designed peptides had a tendency to form an α-helical structure in membrane-mimetic environments. 设计的肽对革兰氏阴性菌表现出有效的抗菌活性且毒性低,在膜模拟环境中倾向于形成α螺旋结构。Among them, peptide LRpG (X: L, zz: pG) showed the highest geometric mean average treatment index (GMTI = 73.1), better salt, temperature and pH stability, and an additive effect with conventional antibiotics. 其中,肽LRpG(X:L,zz:pG)表现出最高的几何平均治疗指数(GMTI = 73.1),更好的盐、温度和pH稳定性,与常规抗生素具有相加作用。Peptide LRpG played the role of anti-Gram-negative bacteria through destroying the cell membrane. In addition, peptide LRpG also exhibited an anti-inflammatory activity by effectively neutralizing endotoxin. 肽LRpG通过破坏细胞膜发挥抗革兰氏阴性菌的作用。此外,肽 LRpG 还通过有效中和内毒素而表现出抗炎活性。Briefly, peptide LRpG has the potential to serve as a therapeutic agent to reduce antibiotic resistance owing to its high therapeutic index and great stability.简而言之,肽 LRpG 由于其高治疗指数和出色的稳定性,有可能作为减少抗生素耐药性的治疗剂。
Keywords: antimicrobial peptides; cell selectivity; stability; mechanism; endotoxin neutralization关键词:抗菌肽;细胞选择性;稳定;机制;内毒素中和
1. Introduction一、简介
With the widespread use of antibiotics in clinical practice in recent years, the resistance rate of bacteria to traditional antibiotics is increasing. The development of antibacterial reagents with new antibacterial mechanisms is imminent [1]. As an important part of innate immunity, antimicrobial peptides (AMPs) form the first line of defense against antimicrobial infections, as found in many microorganisms including insects, plants, and animals [2]. Furthermore, AMPs are known for their anti-inflammatory properties, anti-biofilm formation, promotion of tissue and injury repair, and anti-cancer effects [234]. Compared to traditional antibiotics, AMPs exert an antibacterial effect through non-receptor-mediated membrane permeability. AMPs are expected to be alternatives to antibiotics because of their unique action mechanism [567]. Although more than 3000 AMPs were found, their application as therapeutic agents is seriously hindered due to some limiting factors including manufacturing costs, cytotoxicity, and poor stability [8].近年来,随着抗生素在临床的广泛使用,细菌对传统抗生素的耐药率不断增加。开发具有新抗菌机制的抗菌试剂迫在眉睫[1]。作为先天免疫的重要组成部分,抗菌肽 (AMP) 构成了抵抗抗菌感染的第一道防线,在包括昆虫、植物和动物在内的许多微生物中都有发现 [2]。此外,AMP 以其抗炎特性、抗生物膜形成、促进组织和损伤修复以及抗癌作用而闻名[234]。与传统抗生素相比,AMPs通过非受体介导的膜通透性发挥抗菌作用。由于其独特的作用机制,AMP 有望成为抗生素的替代品[567]。尽管发现了超过3000种AMP,但由于制造成本、细胞毒性和稳定性差等限制因素,它们作为治疗药物的应用受到严重阻碍[8]。
The heptad repeat sequence is a repeat sequence composed of seven amino acids, where the “a” and “d” positions are occupied by hydrophobic amino acids [9]. It was found that natural AMPs possessing heptad repeat sequences have great antibacterial and anti-inflammatory activity, but their application is hindered by high cytotoxicity [1011]. Previous studies mostly focused on how different hydrophobic amino acids at the “a” and “d” positions influence antibacterial activity and cytotoxicity of AMPs, but few studies used this sequence element to design new AMPs [1213]. Some papers showed that symmetric AMPs centered on Gly–Gly (GG) or d-Pro–Gly (pG) have good antibacterial activity and high cell selectivity [14151617]. Therefore, we wondered if the same effect could be achieved when GG or pG was introduced into two symmetric heptad repeat sequences.七肽重复序列是由七个氨基酸组成的重复序列,其中“a”和“d”位置被疏水性氨基酸占据[9]。研究发现,具有七肽重复序列的天然AMP具有很强的抗菌和抗炎活性,但其应用受到高细胞毒性的阻碍[1011]。先前的研究主要集中在“a”和“d”位置的不同疏水性氨基酸如何影响AMP的抗菌活性和细胞毒性,但很少有研究利用该序列元件来设计新的AMP[1213]。一些论文表明,以 Gly-Gly (GG) 或 d-Pro-Gly (pG) 为中心的对称 AMP 具有良好的抗菌活性和高细胞选择性 [14151617]。因此,我们想知道当GG或pG被引入到两个对称的七肽重复序列中时是否可以达到相同的效果。
In this study, two symmetric heptad repeat sequences were connected by a short loop, and the sequence was designed as XXRXXRRzzRRXXRXX-NH2, where X represents Phe (F), Ile (I), and Leu (L), while zz represents Gly–Gly (GG) or d-Pro–Gly (pG). 本研究中,两个对称七肽重复序列通过短环连接,序列设计为XXRXXRRzzRRXXRXX-NH2,其中X代表Phe(F)、Ile(I)和Leu(L),而zz代表Gly–甘氨酸 (GG) 或 d-Pro-甘氨酸 (pG)。The net charge was +6 and the hydrophobicity was 40%–60%, which is consistent with the statistical information on natural AMPs [218]. Hydrophobic amino acids F, I, and L were used to damage microbial cell membranes [19]. Flexible (GG) and rigid (pG) loops were selectively inserted into the middle of two symmetric heptad repeat sequences to enhance cell selectivity [1720]. In addition, Arg (R) was selectively introduced into the sequence template. 净电荷为+6,疏水性为40%~60%,与天然AMPs的统计信息一致[218]。疏水性氨基酸 F、I 和 L 用于损伤微生物细胞膜 [19]。柔性(GG)和刚性(pG)环被选择性地插入两个对称七肽重复序列的中间,以增强细胞选择性[1720]。此外,Arg(R)被选择性地引入到序列模板中。The guanidine side chain of R allows it to form strong bidentate H-bonds with the phosphate moiety of lipid head groups, thereby enhancing the electrostatic action between peptides and anionic bacterial membranes [20]. R 的胍侧链使其能够与脂质头基的磷酸部分形成强的二齿氢键,从而增强肽和阴离子细菌膜之间的静电作用[20]。To determine the role of loops in the designed peptides, a control peptide was designed as a heptad repeat sequence as follows: the central pG loop of peptide LRpG with highest cell selectivity was removed, and Leu located at the N-terminus and C-terminus of the peptide was exchanged with Gly and d-Pro, respectively. 为了确定环在设计的肽中的作用,将对照肽设计为七肽重复序列,如下:去除具有最高细胞选择性的肽LRpG的中央pG环,并且Leu位于N端和C端肽分别与 Gly 和 d-Pro 交换。The positive charge and hydrophobicity were consistent with LRpG. Finally, by amidating the C-terminus of the peptides, the charge of the AMPs was increased, and the stability of the structure was improved [212223]. Using this method of designing and optimizing AMPs, we obtained LRpG (X: L, zz: pG) with high cell selectivity and good conditional stability. 正电荷和疏水性与LRpG一致。最后,通过对肽的C端进行酰胺化,增加了AMP的电荷,提高了结构的稳定性[212223]。利用这种设计和优化 AMP 的方法,我们获得了具有高细胞选择性和良好条件稳定性的 LRpG (X: L, zz: pG)。The antibacterial mechanism study demonstrated that LRpG played the role of an anti-Gram-negative bacterial agent through destroying the cell membrane.抗菌机制研究表明,LRpG通过破坏细胞膜发挥抗革兰氏阴性菌的作用。
2. Results2. 结果
2.1. Peptide Design and Characteristics
In this study, two symmetric heptad repeat sequences were connected by a short loop, and the sequence was designed as XXRXXRRzzRRXXRXX-NH2 (X represents F, I, and L; zz represents GG or pG). 在本研究中,两个对称七肽重复序列通过短环连接,序列设计为XXRXXRRzzRRXXRXX-NH2(X代表F、I和L;zz代表GG或pG)。The molecular mass of all peptides was consistent with theoretical values through measurement, demonstrating that the peptides were successfully synthesized. The hydrophobicity order of the designed peptides was as follows: IRpG > FRpG > IRGG > FRGG = LRpG = LRα > LRGG (Table 1). MALDI-TOF MS of the designed peptides is shown in Supplementary Figure S1, and HPLC spectra of the designed peptides is shown in Supplementary Figure S2.经测定,所有肽的分子量与理论值一致,证明肽合成成功。设计的肽的疏水性顺序如下:IRpG > FRpG > IRGG > FRGG = LRpG = LRα > LRGG(表1)。设计肽的 MALDI-TOF MS 如补充图 S1 所示,设计肽的 HPLC 谱如补充图 S2 所示。
Table 1. Peptides and their key physicochemical parameters.表 1. 肽及其关键理化参数。
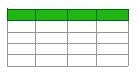
2.2. Circular Dichroism (CD) Spectroscopy
The secondary structure of the designed peptides was detected using CD spectra in 10 mM phosphate-buffered saline (PBS), 50% trifluoroethanol (TFE), and 30 mM sodium dodecyl sulfate (SDS). 使用 CD 光谱在 10 mM 磷酸盐缓冲盐水 (PBS)、50% 三氟乙醇 (TFE) 和 30 mM 十二烷基硫酸钠 (SDS) 中检测设计的肽的二级结构。In 10 mM PBS, the six designed peptides containing a central loop exhibited disordered conformations, and the control LRα showed a more ordered conformation. 在 10 mM PBS 中,设计的六种含有中心环的肽表现出无序构象,而对照 LRα 显示出更有序的构象。In 50% TFE (mimicking the hydrophobic environment of the microbial membrane) and 30 mM SDS (environment comparable to a negatively charged prokaryotic membrane) [15], FRGG, FRpG, IRGG, and IRpG showed disordered conformations. LRpG and LRGG had a tendency to form an α-helical structure with negative peaks at approximately 205 nm and 220 nm. 在 50% TFE(模拟微生物膜的疏水环境)和 30 mM SDS(与带负电荷的原核生物膜相当的环境)中 [15],FRGG、FRpG、IRGG 和 IRpG 显示无序构象。 LRpG 和 LRGG 倾向于形成 α 螺旋结构,负峰位于约 205 nm 和 220 nm 处。In contrast, although the hydrophobic and net charges of the control peptide LRα were consistent with LRpG, a typical α-helical structure with negative peaks at approximately 208 nm and 222 nm was observed (Figure 1). The results reveled that the central loop (pG or GG) disrupted the formation of the α-helical structure. The helical wheel projections of LRGG, LRpG, and LRα are shown in Supplementary Figure S3.相反,虽然对照肽LRα的疏水性和净电荷与LRpG一致,但观察到典型的α螺旋结构,在约208 nm和222 nm处具有负峰(图1)。结果表明,中央环(pG 或 GG)破坏了 α 螺旋结构的形成。 LRGG、LRpG 和 LRα 的螺旋轮投影如补充图 S3 所示。
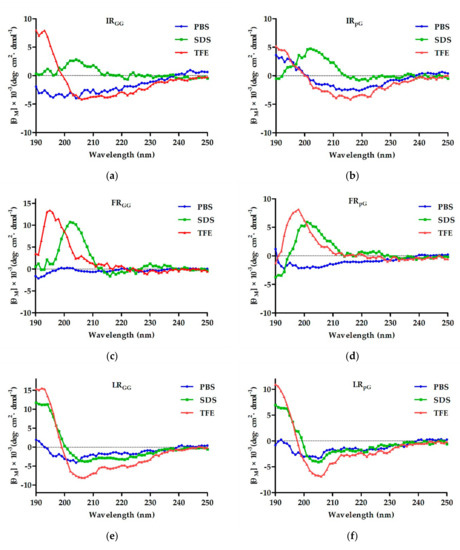
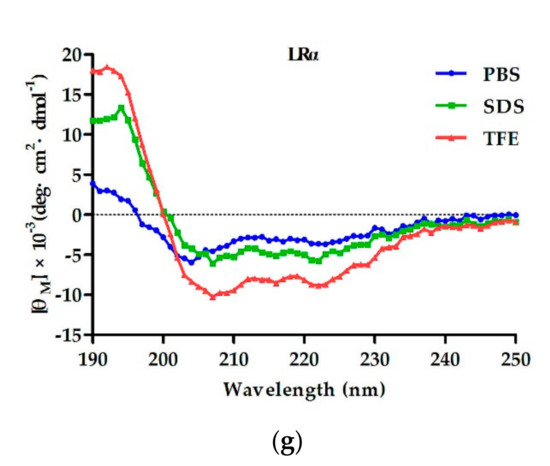
Figure 1. The circular dichroism (CD) spectra of (a) IRGG, (b) IRpG, (c) FRGG, (d) FRpG, (e) LRGG, (f) LRpG, and (g) LRα were dissolved in 10 mM phosphate-buffered saline (PBS) (blue), 30 mM sodium dodecyl sulfate (SDS) (green), and 50% trifluoroethanol (TFE) (red). The average value after three scans of every sample is shown. The CD spectrum of the buffer was subtracted.图 1. (a) IRGG、(b) IRpG、(c) FRGG、(d) FRpG、(e) LRGG、(f) LRpG 和 (g) LRα 溶解在 10 中的圆二色性 (CD) 光谱mM 磷酸盐缓冲盐水 (PBS)(蓝色)、30 mM 十二烷基硫酸钠 (SDS)(绿色)和 50% 三氟乙醇 (TFE)(红色)。显示每个样品三次扫描后的平均值。减去缓冲液的 CD 谱。
2.3. Antimicrobial Activity2.3.抗菌活性
The antimicrobial activity of designed peptides is summarized in Table 2. The designed peptides showed better antimicrobial activity against Gram-negative bacteria than Gram-positive bacteria. 设计的肽的抗菌活性总结于表2中。设计的肽对革兰氏阴性菌的抗菌活性优于对革兰氏阳性菌的抗菌活性。For Gram-negative bacteria, LRpG showed the best antimicrobial activity among the six designed peptides containing a central loop (LRpG > LRGG > FRpG > FRGG > IRpG > IRGG), and the lowest geometric mean minimal inhibitory concentration (GMMIC) value of 3.5 μM. 对于革兰氏阴性菌,LRpG 在六种设计的含有中心环的肽中表现出最佳的抗菌活性(LRpG > LRGG > FRpG > FRGG > IRpG > IRGG),并且几何平均最小抑制浓度(GMMIC)值最低为 3.5 μM 。The control peptide LRα exhibited antimicrobial activity that was a little better than LRpG with a GMMIC value of 3 μM. For Gram-positive bacteria, peptides LRZZ and FRZZ had a relatively weaker antimicrobial effect compared to the control peptide LRα (Table 2).对照肽 LRα 的抗菌活性略优于 LRpG,GMMIC 值为 3 μM。对于革兰氏阳性菌,与对照肽 LRα 相比,肽 LRZZ 和 FRZZ 的抗菌作用相对较弱(表 2)。
Table 2. Minimum inhibitory concentrations (MICs) (µM) and therapeutic index (TI) of the designed peptides.表 2.设计肽的最低抑制浓度 (MIC) (μM) 和治疗指数 (TI)。
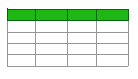
2.4. Biocompatibility Assays
The hemolytic activity of designed peptides against human red blood cells (hRBCs) was determined in a range of 1–512 μM. The MHC10 values of IRGG, IRpG, FRGG, FRpG, LRGG, LRpG, and LRα were 512 μM, 512 μM, 128 μM, 128 μM, 256 μM, 256 μM, and 4 μM, respectively (Figure 2a). In general, the cytotoxicity of the designed peptides was consistent with the hemolytic activity. 设计的肽对人红细胞 (hRBC) 的溶血活性测定范围为 1–512 μM。 IRGG、IRpG、FRGG、FRpG、LRGG、LRpG 和 LRα 的 MHC10 值分别为 512 μM、512 μM、128 μM、128 μM、256 μM、256 μM 和 4 μM(图 2a)。一般来说,设计的肽的细胞毒性与溶血活性一致。Later on, the murine macrophage cell line (RAW 264.7) and human embryonic kidney cells (HEK293T) were treated with 64 μM peptides, and the cell survival rate of RAW 264.7 cells was as follows: IRpG (98%) > IRGG (95%) > LRpG (92%) > LRGG (84%) > FRpG (78%) > FRGG (66%) (Figure 2b). Similarly, the cell survival rate of HEK293T cells was as follows: IRpG (98%) > IRGG (96%) > LRpG (93%) > LRGG (91%) > FRpG (85%) > FRGG (76%) (Supplementary Figure S4). However, the cell survival rate of both cells treated with LRα was less than 10%. To determine the cell selectivity of the designed peptide, the therapeutic index (TI) (MHC10/GMMIC) of each peptide was calculated. 随后,用64 μM肽处理小鼠巨噬细胞系(RAW 264.7)和人胚肾细胞(HEK293T),RAW 264.7细胞的细胞存活率如下:IRpG(98%)> IRGG(95%) ) > LRpG (92%) > LRGG (84%) > FRGG (78%) > FRGG (66%)(图 2b)。同样,HEK293T细胞的细胞存活率如下:IRpG(98%)> IRGG(96%)> LRpG(93%)> LRGG(91%)> FRpG(85%)> FRGG(76%)(补充图S4)。然而,用LRα处理的两种细胞的细胞存活率均低于10%。为了确定设计的肽的细胞选择性,计算了每种肽的治疗指数(TI)(MHC10/GMMIC)。Among all tested peptides, LRpG showed the highest GMTI (73.1) against Gram-negative bacteria (Table 2).在所有测试的肽中,LRpG 针对革兰氏阴性菌表现出最高的 GMTI (73.1)(表 2)。
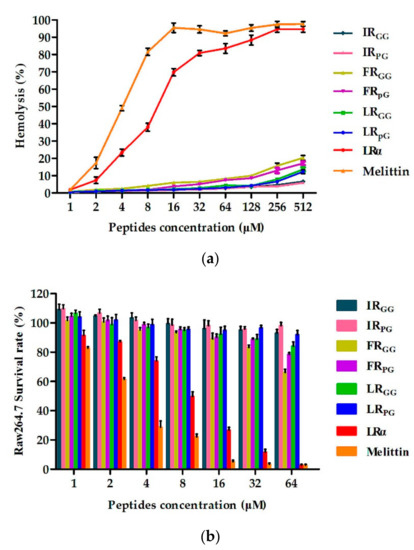
Figure 2. (a) Hemolytic activity of designed peptides against human red blood cells (hRBCs); (b) cytotoxicity of the designed peptides against RAW 264.7 cells. The diagrams are based on at least three independent experiments.图 2. (a) 设计的肽针对人红细胞 (hRBC) 的溶血活性; (b) 设计的肽针对 RAW 264.7 细胞的细胞毒性。这些图表基于至少三个独立实验。
2.5. Condition Sensitivity Assays
These results demonstrated that LRpG could maintain antimicrobial activity in different conditions. Therefore, LRpG may have good potential in clinical application (Table 3 and Table 4).这些结果表明 LRpG 在不同条件下都能保持抗菌活性。因此,LRpG 在临床应用中可能具有良好的潜力(表 3 和表 4)。
Table 3. The MICs (µM) of the designed peptides against E. coli ATCC25922 in the presence of physiological salts.表 3. 在生理盐存在下,设计的肽针对大肠杆菌 ATCC25922 的 MIC (μM)。
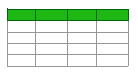
Table 4. The MICs (µM) of the designed peptides against E. coli ATCC25922 after temperature and pH treatment.表 4. 设计的肽在温度和 pH 值处理后针对大肠杆菌 ATCC25922 的 MIC (μM)。
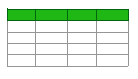
2.6. Additive Effect of AMPs and Conventional Antibiotics2.6。 AMP 与常规抗生素的相加效应
Based on the MICs of the designed peptides and antibiotics, the antimicrobial interactions of peptides and antibiotics were determined using a checkerboard assay. The combination of LRpG and streptomycin exerted a synergistic effect against Escherichia coli ATCC25922. The fractional inhibitory concentration index (FICI) value was 0.5, while the combination of LRpG with other conventional antibiotics produced an additive effect with FICI values from 0.625 to 1 (Table 5).根据设计的肽和抗生素的 MIC,使用棋盘测定法确定肽和抗生素的抗菌相互作用。 LRpG 和链霉素的组合对大肠杆菌 ATCC25922 发挥协同作用。分数抑制浓度指数 (FICI) 值为 0.5,而 LRpG 与其他常规抗生素的组合产生了相加效应,FICI 值为 0.625 至 1(表 5)。
Table 5. The fractional inhibitory concentration index (FICI) 1 for LRpG in combination with conventional antibiotics against E. coli ATCC25922.表 5. LRpG 与常规抗生素组合对抗大肠杆菌 ATCC25922 的分数抑制浓度指数 (FICI) 1。

2.7. Antimicrobial Mechanism Study2.7.抗菌机制研究
2.7.1. Outer Membrane Permeability Assay2.7.1.外膜渗透性测定
Most AMPs kill bacteria by destroying the bacterial cell membrane. Since the outer membrane of the cell is negatively charged at physiological pH, the permeability of the cationic AMPs to the outer membrane is increased [21]. The permeability of peptides to the Gram-negative bacterial outer membrane can be reflected by N-phenyl-1-naphthylamine (NPN) uptake [151721]. NPN is normally excluded from the outer membrane; however, once the outer membrane is permeabilized, NPN is taken up intracellularly and shows increased fluorescence. As shown in Figure 3, LRpG and LRα were able to permeabilize the outer membrane of E. coli ATCC25922 at concentrations from 1 to 32 μM in a concentration-dependent manner. At peptide concentrations greater than 8 µM, the outer membrane permeability induced by LRpG and LRα was over 75%. 大多数 AMP 通过破坏细菌细胞膜来杀死细菌。由于细胞外膜在生理pH值下带负电荷,因此阳离子AMP对外膜的通透性增加[21]。 N-苯基-1-萘胺 (NPN) 的摄取可以反映肽对革兰氏阴性细菌外膜的渗透性 [151721]。 NPN 通常被排除在外膜之外;然而,一旦外膜被透化,NPN 就会被细胞内吸收并显示出增强的荧光。如图 3 所示,LRpG 和 LRα 能够以浓度依赖性方式在 1 至 32 μM 的浓度下透化大肠杆菌 ATCC25922 的外膜。当肽浓度大于 8 µM 时,LRpG 和 LRα 诱导的外膜通透性超过 75%。Moreover, the outer membrane permeability induced by LRα was slightly stronger than that induced by LRpG at the same concentration.此外,相同浓度下,LRα 诱导的外膜通透性略强于 LRpG 诱导的外膜通透性。
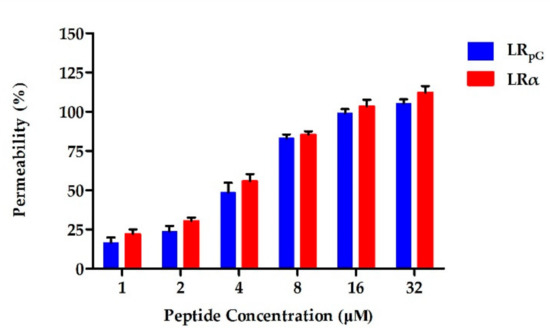
Figure 3. Outer membrane permeability of LRpG and LRα at the concentrations from 1 to 32 μM. The diagram is based on at least three independent experiments.图 3. 浓度为 1 至 32 μM 时 LRpG 和 LRα 的外膜通透性。该图基于至少三个独立实验。
2.7.2. Inner Membrane Permeability Assay
The LRpG permeability to the Gram-negative bacterial inner membrane was determined by measuring the cytoplasm β-galactosidase activity. LRpG and LRα were able to induce an increase in the inner membrane permeability from 0 to 40 min. 通过测量细胞质 β-半乳糖苷酶活性来确定 LRpG 对革兰氏阴性细菌内膜的渗透性。 LRpG 和 LRα 能够诱导 0 至 40 分钟内膜通透性增加。However, LRα displayed an optical density value at 420 nm (OD420) much higher than LRpG at the same point in time. This phenomenon was different from the results of outer membrane permeability (Figure 4).然而,LRα 在 420 nm 处显示的光密度值 (OD420) 远高于同一时间点 LRpG。这种现象与外膜通透性的结果不同(图4)。

Figure 4. Inner membrane permeability of (a) LRpG and (b) LRα at different concentrations. The diagrams are based on at least three independent experiments.图 4. (a) LRpG 和 (b) LRα 在不同浓度下的内膜通透性。这些图表基于至少三个独立实验。
2.7.3. Cytoplasmic Membrane Depolarization
In addition to inner and outer membrane permeability, changes in the plasma membrane potential energy of E. coli ATCC25922 were determined using 3,3’-dipropylthiadicarbocyanine (diSC3-5). The depolarization ability of cell membranes induced by LRpG and LRα was dose- and time-dependent over 1500 s of measured time. 除了内膜和外膜渗透性之外,还使用 3,3'-二丙基硫二碳花青 (diSC3-5) 测定大肠杆菌 ATCC25922 质膜势能的变化。 LRpG 和 LRα 诱导的细胞膜去极化能力在 1500 秒的测量时间内呈剂量和时间依赖性。This experiment showed that LRα had a faster and stronger depolarization ability than LRpG (Figure 5).该实验表明,LRα 比 LRpG 具有更快、更强的去极化能力(图 5)。
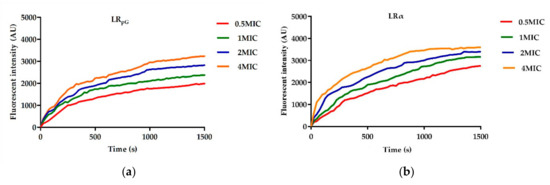
Figure 5. Cytoplasmic membrane potential variation of E. coli ATCC25922 treated with (a) LRpG and (b) LRα at different levels of concentration. The diagram is based on at least three independent experiments.图 5. 用不同浓度水平的 (a) LRpG 和 (b) LRα 处理的大肠杆菌 ATCC25922 的细胞质膜电位变化。该图基于至少三个独立实验。
2.7.4. Scanning Electron Microscopy (SEM)
The cell morphology and membrane damage of E. coli ATCC25922 after LRpG and melittin treatment were observed by SEM. In the control group, E. coli cells had a smooth and bright surface (Figure 6a), while those treated with peptides showed a rough surface with blebbing, as well as shrunken and destroyed shapes. The effect observed in the SEM micrographs was stronger in the cells treated with LRpG than with the control (Figure 6b,c).通过SEM观察LRpG和蜂毒肽处理后大肠杆菌ATCC25922的细胞形态和膜损伤。在对照组中,大肠杆菌细胞具有光滑明亮的表面(图 6a),而用肽处理的细胞则显示出粗糙的表面,有起泡以及收缩和破坏的形状。在 SEM 显微照片中观察到的效果在用 LRpG 处理的细胞中比用对照处理的细胞更强(图 6b、c)。
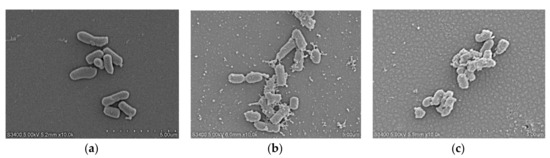
Figure 6. SEM micrographs of E. coli ATCC25922: (a) control; (b) LRpG-treated; (c) LRα-treated.
2.7.5. DNA Binding Assay
The antibacterial effects of AMPs are exerted not only by acting on cell membranes, but also by binding to intracellular substances, such as DNA. Therefore, the ability to induce intracellular effects was measured using a DNA binding analysis. As shown in Figure 7, LRpG and LRα exhibited binding ability at 64 μM and 16 μM, respectively. This experiment showed that LRα had a stronger DNA binding ability than LRpG.AMP的抗菌作用不仅通过作用于细胞膜来发挥,还通过与细胞内物质(例如DNA)结合来发挥。因此,使用 DNA 结合分析来测量诱导细胞内效应的能力。如图 7 所示,LRpG 和 LRα 分别在 64 μM 和 16 μM 时表现出结合能力。本实验表明LRα比LRpG具有更强的DNA结合能力。
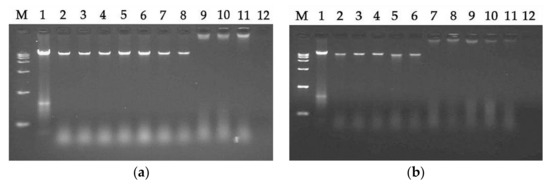
Figure 7. A gel retardation experiment was used to measure the DNA binding assay: (a) LRpG-treated; (b) LRα-treated. 图 7. 使用凝胶阻滞实验来测量 DNA 结合测定:(a) LRpG 处理; (b) LRα 处理。M: DNA marker alone; 1: genomic DNA alone; 2: with 0.5 μM peptide; 3: with 1 μM peptide; 4: with 2 μM peptide; 5: with 4 μM peptide; 6: with 8 μM peptide; 7: with 16 μM peptide; 8: with 32 μM peptide; 9: with 64 μM peptide; 10: with 128 μM peptide; 11: with 256 μM peptide; 12: 256 μM peptide alone.M:单独的DNA标记; 1:单独的基因组DNA; 2:含0.5μM肽; 3:含1μM肽; 4:含2μM肽; 5:含4μM肽; 6:含8μM肽; 7:具有16μM肽; 8:含32μM肽; 9:含有 64 μM 肽; 10:具有128μM肽; 11:含有 256 μM 肽; 12:单独的 256 μM 肽。
2.7.6. Lipopolysaccharide (LPS) Binding Assay
To detect the binding ability between peptides and LPS, the secondary structure of the peptides was measured using the CD spectrum in different concentrations of LPS. As shown in Figure 8, in 10 mM PBS without LPS solution, LRpG exhibited disordered conformations and LRα exhibited a more ordered conformation. However, with the increase in LPS concentration, LRpG and LRα tended to gradually form an α-helical structure. 为了检测肽与LPS之间的结合能力,利用CD光谱在不同浓度的LPS下测量肽的二级结构。如图 8 所示,在不含 LPS 溶液的 10 mM PBS 中,LRpG 表现出无序构象,而 LRα 表现出更有序的构象。然而,随着LPS浓度的增加,LRpG和LRα趋于逐渐形成α螺旋结构。At the molar ratio peptide/LPS of 1:1, both LRpG and LRα began forming an α-helical structure, indicating a similar binding ability to LPS.当肽/LPS摩尔比为1:1时,LRpG和LRα都开始形成α螺旋结构,表明与LPS具有相似的结合能力。
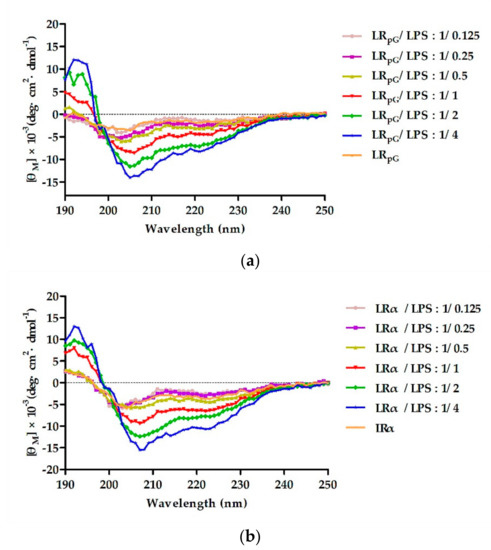
Figure 8. The circular dichroism (CD) spectra of (a) LRpG and (b) LRα were dissolved in different concentrations of lipopolysaccharide (LPS), and the peptide/LPS molar ratio ranged from 1/0.125 to 1/4. The average value after three scans of every sample is shown. The CD spectrum of LPS was subtracted.图 8. (a) LRpG 和 (b) LRα 溶解在不同浓度的脂多糖 (LPS) 中的圆二色性 (CD) 光谱,肽/LPS 摩尔比范围为 1/0.125 至 1/4。显示每个样品三次扫描后的平均值。减去LPS的CD谱。
2.7.7. Limulus Amoebocyte Lysate (LAL) Assay
The chromogenic limulus amoebocyte lysate (LAL) assay was used to analyze the binding ability of the peptide to lipopolysaccharide (LPS). As shown in Figure 9, the activation of the LPS-mediated LAL coagulase was effectively inhibited by both LRpG and LRα in a concentration-dependent manner. 使用显色鲎变形细胞裂解物 (LAL) 测定法分析肽与脂多糖 (LPS) 的结合能力。如图 9 所示,LRpG 和 LRα 以浓度依赖性方式有效抑制 LPS 介导的 LAL 凝固酶的激活。At low concentrations, the binding ability of LRpG to LPS was much stronger than LRα, but the difference tended to be diminished with the increase in peptide concentration.在低浓度下,LRpG 与 LPS 的结合能力比 LRα 强得多,但随着肽浓度的增加,这种差异趋于减小。
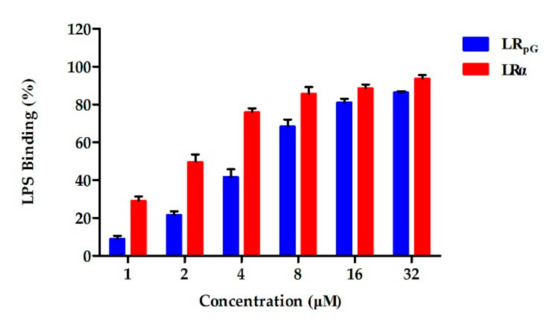
Figure 9. The binding ability of LRpG and LRα to lipopolysaccharide (LPS), determined by limulus amoebocyte lysate (LAL) assay. The diagram is based on at least three independent experiments.图 9. 通过鲎变形细胞裂解物 (LAL) 测定确定 LRpG 和 LRα 与脂多糖 (LPS) 的结合能力。该图基于至少三个独立实验。
2.7.8. Endotoxin Neutralization Assay
To detect the inhibitory effect of LRpG on the inflammatory response induced by LPS, the expression levels of two major inflammatory cytokines, tumor necrosis factor α (TNF-α) and nitric oxide (NO), in the macrophage supernatant were measured. 为了检测 LRpG 对 LPS 诱导的炎症反应的抑制作用,测量了巨噬细胞上清液中两种主要炎症细胞因子肿瘤坏死因子 α (TNF-α) 和一氧化氮 (NO) 的表达水平。Cells without stimulation and cells stimulated with only LPS (100 ng·mL−1) were used as negative and positive controls, respectively. As can be seen from Figure 10, LRpG restrained the production of NO and TNF-α in a concentration-dependent manner and exhibited a significant inhibitory effect at 16 μM. The results showed that LRpG had a good neutralization ability in LPS.未刺激的细胞和仅用LPS(100 ng·mL−1)刺激的细胞分别用作阴性和阳性对照。从图10可以看出,LRpG以浓度依赖性方式抑制NO和TNF-α的产生,并且在16μM时表现出显着的抑制效果。结果表明,LRpG 对 LPS 具有良好的中和能力。

Figure 10. Effects of LRpG on the production of (a) nitric oxide (NO) and (b) tumor necrosis factor α (TNF-α) in LPS-stimulated RAW 264.7 cells. The diagrams are based on the average of three independent experiments. ** p < 0.01, compared to the positive group (only LPS-stimulated).图 10.LRpG 对 LPS 刺激的 RAW 264.7 细胞中 (a) 一氧化氮 (NO) 和 (b) 肿瘤坏死因子 α (TNF-α) 产生的影响。这些图表基于三个独立实验的平均值。 ** p < 0.01,与阳性组(仅 LPS 刺激)相比。
3. Discussion三、讨论
Most AMPs have unique mechanisms of killing bacteria, mainly involving membrane breaking, which endows them with the potential to be alternatives to antibiotics [2]. However, the progress of natural AMPs for therapeutic application is seriously hindered by their inherent defects (such as manufacturing costs, cytotoxicity, poor stability etc.) [28]. To overcome these inherent shortcomings, in this study, six symmetric heptad repeat sequences were connected by a short loop, and the sequence was designed as XXRXXRRzzRRXXRXX-NH2 (X represents F, I, and L; zz represents GG or pG).大多数AMP具有独特的杀菌机制,主要涉及破膜,这使得它们有可能成为抗生素的替代品[2]。然而,天然AMPs的治疗应用进展受到其固有缺陷(如制造成本、细胞毒性、稳定性差等)的严重阻碍[28]。为了克服这些固有的缺点,在本研究中,六个对称七肽重复序列通过短环连接,序列设计为XXRXXRRzzRRXXRXX-NH2(X代表F、I和L;zz代表GG或pG)。
The results of MICs indicated that the designed peptides had good antibacterial activity toward Gram-negative bacteria (Table 2). Compared to the single membrane and thick peptidoglycan layer of Gram-positive bacteria, the double-membrane structure of Gram-negative bacteria represents a weaker barrier; thus, Gram-negative bacteria are more sensitive to AMPs [172124]. The antibacterial activity of AMPs can be affected by many factors. MIC结果表明,设计的肽对革兰氏阴性菌具有良好的抗菌活性(表2)。与革兰氏阳性菌的单膜和厚肽聚糖层相比,革兰氏阴性菌的双膜结构代表了较弱的屏障;因此,革兰氏阴性细菌对 AMP 更敏感 [172124]。 AMP 的抗菌活性会受到多种因素的影响。Previous studies demonstrated that a proper positive charge (+6 to +7) was essential for antibacterial activity, but antibacterial activity was no longer increased when the positive charge of AMPs was beyond the threshold [1720]. In this study, the positive charge of the designed peptides was set to +6. Based on their hydrophobicity, the theoretical antibacterial activity of the designed peptides would be IRZZ > FRZZ > LRZZ = LRα; however, the result was LRα > LRZZ > FRZZ > IRZZ. 先前的研究表明,适当的正电荷(+6至+7)对于抗菌活性至关重要,但当AMP的正电荷超过阈值时,抗菌活性不再增加[1720]。在本研究中,设计的肽的正电荷设置为+6。根据其疏水性,设计的肽的理论抗菌活性为 IRZZ > FRZZ > LRZZ = LRα;然而,结果是 LRα > LRZZ > FRZZ > IRZZ。Combined with the results of CD, although both LRZZ and LRα had the tendency to form an α-helical structure in membrane-mimetic environments, LRα’s tendency was stronger. One possible explanation is that the rigid loop (pG) of LRZZ disrupted the α-helical structure. 结合CD的结果,虽然LRZZ和LRα在拟膜环境中都有形成α螺旋结构的倾向,但LRα的倾向更强。一种可能的解释是 LRZZ 的刚性环 (pG) 破坏了 α 螺旋结构。Furthermore, we found that both FRZZ and IRZZ exhibited disordered conformations in membrane-mimetic environments. 此外,我们发现 FRZZ 和 IRZZ 在膜模拟环境中都表现出无序构象。The result further confirmed the previous perspectives that the antibacterial activity of designed peptides is not only connected to the hydrophobicity of the peptides but also to the spatial stability of the secondary structure [25]. In addition, the antibacterial activity of XRpG was higher than that of XRGG. Some papers confirmed that the rigid loop (pG), which promotes tightness in the hydrophobic center, has a better effect than the flexible loop (GG) [20].结果进一步证实了之前的观点,即设计的肽的抗菌活性不仅与肽的疏水性有关,还与二级结构的空间稳定性有关[25]。此外,XRpG 的抗菌活性高于 XRGG。有论文证实,促进疏水中心紧密度的刚性环(pG)比柔性环(GG)效果更好[20]。
However, the antimicrobial activity of AMPs is not the only criterion when evaluating their potential for clinical application. The hemolytic activity and cytotoxicity to mammalian cells are also major limiting factors [2627]. Therefore, the cytotoxicity and hemolytic activity of the designed peptides were tested. At all examined concentrations, the designed peptides had much lower hemolysis and cytotoxicity (Figure 2), showing better cell selectivity compared to LRα. This result may be due to the insertion of the central loop as previous papers reported. 然而,AMP 的抗菌活性并不是评估其临床应用潜力的唯一标准。对哺乳动物细胞的溶血活性和细胞毒性也是主要的限制因素[2627]。因此,测试了设计的肽的细胞毒性和溶血活性。在所有检查的浓度下,设计的肽具有低得多的溶血性和细胞毒性(图 2),与 LRα 相比,显示出更好的细胞选择性。这一结果可能是由于先前论文报道的中央循环的插入所致。The cell selectivity of XRpG was better than that of XRGG, which may be due to the rigid pG having a better effect on promoting cell selectivity than flexible GG [20]. As shown in Table 2, LRpG had a great balance between antibacterial activity and cytotoxicity, and it had higher cell selectivity.XRpG 的细胞选择性优于 XRGG,这可能是由于刚性 pG 比柔性 GG 具有更好的促进细胞选择性的效果 [20]。如表2所示,LRpG在抗菌活性和细胞毒性之间具有很好的平衡,并且具有较高的细胞选择性。
In addition to the cell selectivity assays of AMPs, stability is also a major reason impeding AMPs from becoming therapeutic agents [22829]. In this study, the effects of the physiological concentrations of salts, temperature, and pH on the antibacterial activity of the designed peptides were investigated. As shown in Table 3, in salt ions, the antimicrobial activity of LRpG against E. coli ATCC25922 was negligibly affected by all tested cations except for Na+. Previous reports suggested that a stable α-helical structure helps salt tolerance [30]. However, the insertion of the loop (pG) structure into the sequence template still resulted in good salt stability in this study. The result was due to the loop (pG) promoting hydrogen bonding and hydrophobic interactions, thereby achieving the effect of salt tolerance [31]. The antibacterial activity of LRpG against E. coli ATCC25922 decreased because of the disruption of electrostatic interaction between LRpG and the cell membrane in the presence of 150 mM Na+ [32]. For the clinical application of AMPs, thermal stability is very important since many foods and feed products need to be heated during processing [33]. LRpG exerted good thermal stability (Table 4) and could maintain antibacterial activity in different pH conditions.除了 AMP 的细胞选择性测定之外,稳定性也是阻碍 AMP 成为治疗剂的主要原因 [22829]。在这项研究中,研究了盐的生理浓度、温度和pH值对所设计的肽的抗菌活性的影响。如表 3 所示,在盐离子中,LRpG 对大肠杆菌 ATCC25922 的抗菌活性几乎不受除 Na+ 之外的所有测试阳离子的影响。之前的报道表明,稳定的α-螺旋结构有助于耐盐性[30]。然而,在本研究中,将环(pG)结构插入序列模板中仍然产生良好的盐稳定性。结果是由于环(pG)促进氢键和疏水相互作用,从而达到耐盐的效果[31]。由于 150 mM Na+ 存在下 LRpG 与细胞膜之间的静电相互作用被破坏,LRpG 对大肠杆菌 ATCC25922 的抗菌活性降低[32]。对于AMP的临床应用,热稳定性非常重要,因为许多食品和饲料产品在加工过程中需要加热[33]。 LRpG 表现出良好的热稳定性(表 4),并且可以在不同 pH 条件下保持抗菌活性。
Furthermore, LRpG exhibited a synergistic effect with streptomycin and additive effects with other antibiotics against E. coli ATCC25922 (Table 5). Some studies reported that the antibacterial effect of streptomycin and chloramphenicol involved acting on ribosomes, while ciprofloxacin acted on DNA gyrase, and cefotaxime acted on the cell membrane. 此外,LRpG 对大肠杆菌 ATCC25922 表现出与链霉素的协同作用以及与其他抗生素的相加作用(表 5)。有研究报道链霉素和氯霉素的抗菌作用涉及核糖体,环丙沙星作用于DNA旋转酶,头孢噻肟作用于细胞膜。In this study, LRpG acted on cell membranes to increase membrane permeability and promote antibiotic entry into cells [3435]. The combination of LRpG and antibiotics improved the binding efficiency and ultimately enhanced the therapeutic efficacy. Therefore, LRpG can serve as an additive to decrease the dose of antibiotics.在这项研究中,LRpG 作用于细胞膜,增加膜通透性并促进抗生素进入细胞 [3435]。 LRpG 与抗生素的结合提高了结合效率,最终增强了治疗效果。因此,LRpG 可以作为添加剂来减少抗生素的剂量。
In this study, we also compared a natural AMP (melittin) to LRpG in the GMMIC, MHC10, GMSI, and stability results. Although it exhibited a slightly better antibacterial activity than LRpG, melittin had a strong hemolysis effect. 在这项研究中,我们还在 GMMIC、MHC10、GMSI 和稳定性结果中比较了天然 AMP(蜂毒肽)与 LRpG。虽然其抗菌活性比LRpG略好,但蜂毒肽具有很强的溶血作用。By calculating the GMSI values (MHC10/GMMIC), we found that the GMSI of LRpG was 584 times higher than that of melittin. Furthermore, LRpG exerted a good conditional stability and additive effects with conventional antibiotics. 通过计算 GMSI 值 (MHC10/GMMIC),我们发现 LRpG 的 GMSI 比蜂毒肽高 584 倍。此外,LRpG 与传统抗生素相比具有良好的条件稳定性和相加效应。Based on the results above, LRpG overcomes the main limiting factors of natural AMPs (such as melittin) and has the potential to develop into a therapeutic agent. Therefore, we further studied the antibacterial mechanism of LRpG. 基于上述结果,LRpG克服了天然AMP(如蜂毒肽)的主要限制因素,具有开发成为治疗剂的潜力。因此,我们进一步研究了LRpG的抗菌机制。Previous studies demonstrated that cationic AMPs combine with anions on the surface of bacteria via electrostatic action, and their hydrophobic amino acids are inserted into the bacterial cell membrane. 此前的研究表明,阳离子AMP通过静电作用与细菌表面的阴离子结合,其疏水性氨基酸插入细菌细胞膜中。Once the critical concentration is exceeded, the membrane breaks, and this ultimately leads to death of the bacteria [2122]. LRpG firstly bound to the LPS of the outer membrane of E. coli ATCC25922 through electrostatic action (Figure 8), and then destroyed the cell membrane. Compared to the outer membrane, LRpG had a much weaker permeability to the inner membrane than LRα (Figure 3 and Figure 4). This result might be due to the decreased helical tendency of the LRpG as a result of the loop (pG), which was consistent with the previous papers showing that the helical tendency of AMPs was closely related to the permeability of bacterial inner membrane [20]. After breaking the impermeable barrier, LRpG interacted with the cytoplasmic membrane, resulting in pore and ion channel formation (Figure 5). SEM results directly confirmed that LRpG killed E. coli (Figure 6) by destroying the bacterial cell membranes and causing intracellular lysate leakage. In addition to the membrane mechanisms mentioned above, LRpG can bind to intracellular material DNA at a concentration much higher than the MIC (Figure 7), suggesting that LRpG cannot kill bacteria by DNA binding at the MIC. Furthermore, we found that the DNA binding ability of LRpG was relatively weaker than that of LRα. One possible reason is that the α-helical forming ability of LRpG was weaker than that of LRα. 一旦超过临界浓度,膜就会破裂,最终导致细菌死亡[2122]。 LRpG首先通过静电作用与大肠杆菌ATCC25922外膜的LPS结合(图8),然后破坏细胞膜。与外膜相比,LRpG 对内膜的渗透性比 LRα 弱得多(图 3 和图 4)。这一结果可能是由于环(pG)导致LRpG的螺旋倾向降低,这与之前的论文一致,表明AMP的螺旋倾向与细菌内膜的通透性密切相关[20] 。打破不可渗透的屏障后,LRpG 与细胞质膜相互作用,导致孔和离子通道形成(图 5)。 SEM 结果直接证实 LRpG 通过破坏细菌细胞膜并导致细胞内裂解物泄漏来杀死大肠杆菌(图 6)。除了上述膜机制外,LRpG 还可以以远高于 MIC 的浓度与细胞内物质 DNA 结合(图 7),这表明 LRpG 不能通过 MIC 处的 DNA 结合来杀死细菌。此外,我们发现 LRpG 的 DNA 结合能力相对弱于 LRα。一个可能的原因是 LRpG 的 α-螺旋形成能力弱于 LRα。In short, LRpG exerted its antibacterial effect by destroying the integrity of the bacterial cell membrane, causing leakage of the cellular content and binding to DNA through the cell membrane.简而言之,LRpG 通过破坏细菌细胞膜的完整性,导致细胞内容物泄漏并通过细胞膜与 DNA 结合来发挥抗菌作用。
LPS is a crucial component of the outer membrane in Gram-negative bacteria [36]. Following bacterial death under the action of antibacterial agents, a large amount of LPS detaches from the cells and enters into the blood circulation of the body, activates the inflammatory signaling pathway, and releases inflammatory mediators such as NO and TNF-α [3738]. Previous studies showed that positively charged amphipathic AMPs had a strong LPS neutralization ability and were ideal candidates for anti-inflammatory drugs [3940]. The LPS-induced inflammatory mediators NO and TNF-α were remarkably inhibited by LRpG in a concentration-dependent manner (Figure 10). Based on this study, LRpG has the potential to develop into an anti-inflammatory agent by blocking LPS-mediated inflammatory mediators.LPS 是革兰氏阴性细菌外膜的重要组成部分[36]。细菌在抗菌药物作用下死亡后,大量LPS从细胞中脱离进入机体血液循环,激活炎症信号通路,释放NO、TNF-α等炎症介质[3738]。先前的研究表明,带正电荷的两亲性 AMP 具有很强的 LPS 中和能力,是抗炎药物的理想候选者 [3940]。 LPS 诱导的炎症介质 NO 和 TNF-α 被 LRpG 以浓度依赖性方式显着抑制(图 10)。根据这项研究,LRpG 有潜力通过阻断 LPS 介导的炎症介质而发展成为抗炎剂。
4. Materials and Methods4. 材料与方法
4.1. Materials
Escherichia coli (E. coli) ATCC25922, E. coli K88, Pseudomonas aeruginosa (P. aeruginosa) ATCC27853, Salmonella Pullorum (S. Pullorum) NCTC5776, Klebsiella pneumoniae (K. pneumoniae) CMCC46117, Staphylococcus aureus (S. aureus) ATCC25923, S. aureus ATCC29213, methicillin-resistant S. aureus ATCC43300, and Enterococcus faecalis (E. faecalis) ATCC29212 were obtained from the pharmacology and toxicology laboratory of the College of Animal Science and Technology, Jilin Agricultural University (Changchun, China). 大肠杆菌 (E. coli) ATCC25922、大肠杆菌 K88、铜绿假单胞菌 (P. aeruginosa) ATCC27853、沙门氏菌 (S. Pullorum) NCTC5776、肺炎克雷伯菌 (K.肺炎) CMCC46117、金黄色葡萄球菌 (金黄色葡萄球菌)ATCC25923,金黄色葡萄球菌 ATCC29213、耐甲氧西林金黄色葡萄球菌 ATCC43300 和粪肠球菌 (E. faecalis) ATCC29212 获自吉林农业大学动物科技学院药理毒理实验室(中国长春)。HEK293T and RAW 264.7 cells were obtained from the College of Animal Science and Technology, Jilin Agricultural University (Changchun, China). The hRBCs were obtained from the Jilin Agricultural University Hospital.HEK293T和RAW 264.7细胞获自吉林农业大学动物科技学院(中国长春)。 hRBC 购自吉林农业大学医院。
Mueller–Hinton broth (MHB) powder and Mueller–Hinton agar (MHA) were purchased from GL Biochem (Shanghai, China). Bovine serum albumin (BSA), Triton X-100, and phosphate-buffered saline (PBS) solution were purchased from Solarbio (Beijing, China). O-nitrophenyl-β-d-galactopyranoside (ONPG), LPS from E. coli 0111 B4, diSC3-5, polymyxin B, 4-(2-hydroxyethyl) piperazine-1-ethanesulfonic acid (HEPES), and NPN were all purchased from Sigma-Aldrich (Shanghai, China). Mueller-Hinton 肉汤 (MHB) 粉末和 Mueller-Hinton 琼脂 (MHA) 购自 GL Biochem(中国上海)。牛血清白蛋白(BSA)、Triton X-100和磷酸盐缓冲盐水(PBS)购自Solarbio(中国北京)。 O-硝基苯基-β-d-吡喃半乳糖苷 (ONPG)、来自大肠杆菌 0111 B4 的 LPS、diSC3-5、多粘菌素 B、4-(2-羟乙基) 哌嗪-1-乙磺酸 (HEPES) 和 NPN 均购买来自 Sigma-Aldrich(中国上海)。TransDetect Cell Counting Kit-8 (CCK-8) and Dulbecco’s modified Eagle’s medium with high glucose (DMEM) were purchased from TransGen Biotech (Beijing, China). Fetal bovine serum (FBS) was purchased from Gibco (Shanghai, China).TransDetect Cell Counting Kit-8 (CCK-8) 和 Dulbecco 改良 Eagle's 培养基 (DMEM) 购自 TransGen Biotech(中国北京)。胎牛血清(FBS)购自 Gibco(中国上海)。
4.2. Synthesis and Sequence Analysis of Peptides4.2.肽的合成和序列分析
The designed peptides were sent to GL Biochem (Shanghai, China) for synthesis and purification. The purity of the peptide was determined by reverse-phase high-performance liquid chromatography on an analytical Kromasil C18 column (Beijing, China). 设计的肽被送往GL Biochem(中国上海)进行合成和纯化。肽的纯度通过分析 Kromasil C18 柱(中国北京)上的反相高效液相色谱测定。The purity of the peptide was over 95% (RP-HPLC). ESI-MS analysis was used to measure the molecular weight of designed peptides, and values were very close to the calculated values. The primary physical and chemical parameters of the designed peptides were measured online at http://web.expasy.org/compute_pi/. The hydrophobicity and relative hydrophobic moments were measured online at http://heliquest.ipmc.cnrs.fr/.肽的纯度超过95%(RP-HPLC)。使用ESI-MS分析测量了设计的肽的分子量,结果与计算值非常接近。设计的肽的主要物理和化学参数在 http://web.expasy.org/compute_pi/ 上在线测量。疏水性和相对疏水矩在 http://heliquest.ipmc.cnrs.fr/ 上在线测量。
4.3. CD Spectroscopy
The CD Spectroscopy of the designed peptides was detected on a J-810 spectropolarimeter (Jasco, Tokyo, Japan) at 25 °C. The final concentration of peptides in 10 mM PBS (pH 7.4), 50% TFE, and 30 mM SDS was 150 µM. The experimental parameters were as follows: 10 nm·min设计的肽的 CD 光谱在 J-810 分光偏振计(Jasco,东京,日本)上于 25 °C 下检测。 10 mM PBS (pH 7.4)、50% TFE 和 30 mM SDS 中肽的最终浓度为 150 µM。实验参数如下:10 nm·min
−1
scanning rate, 1 mm path length, and 190–250 nm wavelength range. Each peptide was scanned an average of three times. The collected data were transformed to mean residue ellipticity as follows:扫描速率、1 mm 路径长度和 190–250 nm 波长范围。每个肽平均扫描三次。将收集的数据转换为平均残差椭圆率,如下所示:
θM = θobs × 1000l × c × n�M = �obs × 1000l × c × nθM = θobs × 1000l × c × n M = obs × 1000l × c × n
(1)
where θ
M
represents the mean residue ellipticity (deg·cm
2
·dmol
−1
), θ
obs
represents the ellipticity correction of that measurement buffer at a given wavelength (mdeg), l represents the path length (cm), c represents the peptide concentration (mM), and n represents the number of amino residues.表示给定波长 (mdeg) 下测量缓冲液的椭圆度校正,l 表示光程长度 (cm),c 表示肽浓度 (mM),n 表示氨基残基数。
4.4. MIC Measurements
The antimicrobial activity of the designed peptides was examined using the micro-dilution method described previously [29]. The bacteria were cultured for 16 h in MHB at 37 °C, and the microbial suspension was diluted to a final concentration of 105 colony-forming units (CFU)/mL. 使用先前描述的微量稀释方法检查所设计的肽的抗菌活性[29]。将细菌在 37°C 的 MHB 中培养 16 小时,并将微生物悬浮液稀释至终浓度 105 菌落形成单位 (CFU)/mL。Then, 50 µL of MHB containing different concentrations peptides (0.25–256 µM) was mixed with 50 µL of bacterial solution, and the mixture was added to a 96-well plate. 然后,将 50 µL 含有不同浓度肽(0.25–256 µM)的 MHB 与 50 µL 细菌溶液混合,并将混合物添加到 96 孔板中。After incubation for 16 h, MIC was examined by measuring the OD at 492 nm (Microplate reader, TECAN GENios F129004, Tecan, Salzburg, Austria). The assays were performed three times.孵育 16 小时后,通过测量 492 nm 处的 OD 来检查 MIC(酶标仪,TECAN GENios F129004,Tecan,萨尔茨堡,奥地利)。测定进行三次。
4.5. Biocompatibility Assays
The hemolytic activity was examined based on the method mentioned previously [22]. Briefly, the collected hRBCs were washed three times and diluted at 2% (v/v) with PBS (pH 7.4). Then, 100 µL of hRBCs were put into each tube including 100 µL of two-fold diluted peptides. The mixtures were centrifuged at 1000× g for 3 min. The OD value at 570 nm of the supernatant was measured, describing hemoglobin release (Microplate reader, TECAN GENios F129004, Tecan, Salzburg, Austria). 溶血活性检测参照前述方法[22]。简而言之,将收集的 hRBC 洗涤 3 次,并用 PBS (pH 7.4) 稀释至 2% (v/v)。然后,将 100 µL hRBC 放入每个包含 100 µL 两倍稀释肽的管中。将混合物以 1000×g 离心 3 分钟。测量上清液 570 nm 处的 OD 值,描述血红蛋白释放(酶标仪,TECAN GENios F129004,Tecan,萨尔茨堡,奥地利)。For this assay, 1% Triton X-100-treated hRBCs were used as a positive control and untreated hRBCs were used as a negative control. MHC10 was defined as the peptide concentration that caused 10% hemolysis. The assays were performed three times.对于该测定,1% Triton X-100 处理的 hRBC 用作阳性对照,未处理的 hRBC 用作阴性对照。 MHC10 定义为引起 10% 溶血的肽浓度。测定进行三次。
The cytotoxicity was detected according to a CCK-8 assay as described previously. Briefly, RAW 264.7 and HEK293T cells were placed into 96-well plates at a density of 1.0–2.0 × 105, and then cultured for 16 h under conditions of 5% CO2. Then, two-fold diluted peptides in DMEM were added to the plates. 根据先前描述的CCK-8测定法检测细胞毒性。简而言之,将RAW 264.7和HEK293T细胞以1.0-2.0×105的密度放入96孔板中,然后在5%CO2条件下培养16小时。然后,将 DMEM 中两倍稀释的肽添加到板中。After 16 h of culturing, CCK-8 (10%, v/v) was put into each well containing cell culture, and mixtures were cultured for 2 h. The cytotoxicity assay was examined by measuring the OD of the mixtures at 450 nm (Microplate reader, TECAN GENios F129004, Tecan, Salzburg, Austria). The assays were performed three times.培养16小时后,将CCK-8(10%,v/v)放入含有细胞培养物的每个孔中,并将混合物培养2小时。通过在 450 nm 处测量混合物的 OD(酶标仪,TECAN GENios F129004,Tecan,萨尔茨堡,奥地利)来检查细胞毒性测定。测定进行三次。
4.6. Condition Sensitivity Assays
The salt, temperature, and pH sensitivities of designed peptides were determined by MIC assay as described previously [40]. For the salt sensitivity assay, peptides were diluted in deionized water containing different salts, and mixed with E. coli ATCC25922 (105 CFU/mL). The final physiological concentrations of different salts were as follows: 150 mM NaCl, 4.5 mM KCl, 6 μM NH4Cl, 1 mM MgCl2, 8 μM ZnCl2, and 4 μM FeCl3. For temperature sensitivity, peptides were cooled on ice for 10 min, and incubated at 37 °C and 100 °C for 30 min. 如前所述,通过 MIC 测定确定设计肽的盐、温度和 pH 敏感性 [40]。对于盐敏感性测定,将肽在含有不同盐的去离子水中稀释,并与大肠杆菌 ATCC25922 (105 CFU/mL) 混合。不同盐的最终生理浓度如下:150 mM NaCl、4.5 mM KCl、6 μM NH4Cl、1 mM MgCl2、8 μM ZnCl2 和 4 μM FeCl3。为了提高温度敏感性,将肽在冰上冷却 10 分钟,并在 37 °C 和 100 °C 下孵育 30 分钟。For pH sensitivity, peptides were treated with different pH for 1 h. Briefly, peptides were diluted in deionized water, and the pH of the solution was adjusted to 4.0, 6.0, 8.0, and 10.0 with HCl or NaOH. The subsequent steps were the same as for the MIC measurements. 为了提高 pH 敏感性,将肽用不同的 pH 值处理 1 小时。简而言之,将肽在去离子水中稀释,并用HCl或NaOH将溶液的pH调节至4.0、6.0、8.0和10.0。后续步骤与 MIC 测量相同。These tests were performed three times.这些测试进行了三次。
4.7. Synergy with Conventional Antibiotics4.7.与传统抗生素的协同作用
The combined antibacterial effect of peptides with other antibiotics was evaluated by checkerboard assay as described previously [如前所述,通过棋盘测定法评估肽与其他抗生素的联合抗菌作用[
]. Briefly, peptides and antibiotics diluted two-fold were prepared at concentrations ranging from 1/8× MIC to 2× MIC. ]。简而言之,将肽和抗生素稀释两倍,浓度范围为 1/8× MIC 至 2× MIC。Subsequently, each peptide at the same concentration was added to the longitudinal column of wells, and each antibiotic at the same concentration was added to the horizontal row of wells. A suspension at 10随后,将相同浓度的每种肽添加到纵向列的孔中,并将相同浓度的每种抗生素添加到水平行的孔中。 10点暂停
5
CFU/mL was put into each well for 16 h at 37 °C. Each assay was performed three times. The fractional inhibitory concentration (FIC) index was calculated as follows:将 CFU/mL 放入每个孔中,37°C 培养 16 小时。每个测定进行三次。抑制浓度分数(FIC)指数计算如下:
FIC = MIC of drug A in combinationMIC of drug A alone+MIC of drug B in combinationMIC of drug B aloneFIC = MIC of drug A in combinationMIC of drug A alone+MIC of drug B in combinationMIC of drug B aloneFIC = 药物 A 组合中的 MIC 单独药物 A 的 MIC + 组合中药物 B 的 MIC 单独药物 B 的 MIC FIC = 组合中药物 A 的 MIC 单独药物 A 的 MIC + 组合中药物 B 单独的 MIC 单独药物 B 的 MIC
(2)
where FIC ≤ 0.5 denotes synergy, 0.5 < FIC ≤ 1.0 denotes additive, and 1.0 < FIC ≤ 4.0 denotes indifferent.其中 FIC ≤ 0.5 表示协同作用,0.5 < FIC ≤ 1.0 表示相加作用,1.0 < FIC ≤ 4.0 表示无影响。
4.8. Outer Membrane Permeability Assay4.8.外膜渗透性测定
The outer membrane permeability was examined using fluorescent dye NPN according to the method mentioned previously [根据前面提到的方法,使用荧光染料NPN检查外膜通透性[
]. Briefly,
E. coli
ATCC25922 cells were washed three times and diluted to 10ATCC25922细胞洗涤3次并稀释至10
5
CFU/mL in 5 mM HEPES buffer (pH 7.4, containing 5 mM glucose). Then, the final concentration of 10 μM NPN was added to the suspension, and incubated at room temperature in the dark for 30 min. 5 mM HEPES 缓冲液(pH 7.4,含有 5 mM 葡萄糖)中的 CFU/mL。然后,将终浓度为10μM的NPN添加到悬浮液中,并在室温下避光孵育30分钟。An equal volume of suspension and peptides at various concentrations were mixed in a black 96-well plate. The fluorescence intensities of the samples were measured (emission λ = 420 nm, excitation λ = 350 nm) with an F4500 fluorescence spectrophotometer (Hitachi, Tokyo, Japan). 将等体积的悬浮液和不同浓度的肽在黑色 96 孔板中混合。使用 F4500 荧光分光光度计(日本东京日立)测量样品的荧光强度(发射 λ = 420 nm,激发 λ = 350 nm)。Polymyxin B (10 µg/mL) with strong outer membrane permeability was used as a positive control. Values were converted to percentage NPN uptake using the following equation:使用具有强外膜渗透性的多粘菌素 B (10 µg/mL) 作为阳性对照。使用以下公式将值转换为 NPN 吸收百分比:
NPN uptake (%) = Fobs−F0F100−F0×100NPN uptake (%) = Fobs−F0F100−F0×100NPN 摄取量 (%) = Fobs−F0F100−F0×100NPN 摄取量 (%) = Fobs−F0F100−F0×100
(3)
where F
obs
is the observed fluorescence of NPN with是观察到的 NPN 荧光
E. coli
ATCC25922 cells at a given peptide concentration, FATCC25922 细胞在给定的肽浓度下,F
0
is the initial fluorescence of NPN with是 NPN 的初始荧光
E. coli
ATCC25922 cells, and FATCC25922细胞和F
100
is the fluorescence of NPN with是 NPN 的荧光
E. coli
ATCC25922 cells with the addition of 10 µg/mL polymyxin B.添加 10 µg/mL 多粘菌素 B 的 ATCC25922 细胞。
4.9. Inner Membrane Permeability Assay
The inner membrane permeabilization was determined by measuring the cytoplasm β-galactosidase activity according to the method mentioned previously [21]. In short, an equal volume of the suspension of E. coli ATCC25922 cells was washed three times and diluted to an OD600 of 0.05 with 10 mM PBS (containing 1.5 mM ONPG); then, the suspension was put into each well of a 96-well plate and cultured with 0.5× MIC to 4× MIC peptides at 37 °C. 根据前面提到的方法通过测量细胞质β-半乳糖苷酶活性来确定内膜通透性[21]。简而言之,将等体积的大肠杆菌ATCC25922细胞悬液洗涤3次,并用10 mM PBS(含1.5 mM ONPG)稀释至OD600为0.05;然后将悬浮液放入96孔板的每个孔中,与0.5×MIC至4×MIC的肽在37℃下培养。The OD at 420 nm (Microplate reader, TECAN GENios F129004, Tecan, Salzburg, Austria) was recorded for 40 min every 5 min eight times. The method was used to detect the permeabilization of the inner membrane through the reflection of the ONPG influx into the cells.420 nm 处的 OD(酶标仪,TECAN GENios F129004,Tecan,萨尔茨堡,奥地利)每 5 分钟记录 40 分钟八次。该方法用于通过 ONPG 流入细胞的反射来检测内膜的通透性。
4.10. Cytoplasmic Membrane Depolarization Assay
DiSC3-5, a membrane potential-sensitive fluorescent dye, was used to assess the ability of the peptides to disrupt the cytoplasmic membrane as previously described, [42]. Briefly, E. coli ATCC25922 in the mid-log phase was washed three times and diluted to an OD600 of 0.05 with 5 mM HEPES buffer (containing 20 mM glucose and 100 mM KCl); then, the mixture of E. coli ATCC25922 and 0.5× MIC to 4× MIC peptides was mixed with diSC3-5 (final concentration of 0.4 μM) at 37 °C for 1 h. DiSC3-5 是一种膜电位敏感荧光染料,用于评估肽破坏细胞质膜的能力,如前所述 [42]。简而言之,将处于对数中期的大肠杆菌 ATCC25922 洗涤 3 次,并用 5 mM HEPES 缓冲液(含有 20 mM 葡萄糖和 100 mM KCl)稀释至 OD600 为 0.05;然后,将大肠杆菌 ATCC25922 和 0.5×MIC 至 4×MIC 肽的混合物与 diSC3-5(终浓度 0.4 μM)在 37°C 混合 1 小时。The fluorescence changes from 0 to 1500 s were recorded at the emission wavelength (λ = 670 nm) and excitation wavelength (λ = 622 nm) (Fluorescence Spectrophotometer, F4500, Hitachi, Tokyo, Japan).记录发射波长(λ = 670 nm)和激发波长(λ = 622 nm)从 0 到 1500 秒的荧光变化(荧光分光光度计,F4500,日立,东京,日本)。
4.11. Scanning Electron Microscopy4.11.扫描电子显微镜
E. coli ATCC25922 cells in the mid-log phase were washed three times and diluted to 107 CFU/mL in 10 mM PBS. The bacterial suspension and peptide (1× MIC) were added to 24-well plates containing polylysine-treated glass slides. The bacteria untreated by peptide were used as a control. 对数中期大肠杆菌 ATCC25922 细胞洗涤 3 次,并在 10 mM PBS 中稀释至 107 CFU/mL。将细菌悬浮液和肽 (1×MIC) 添加到含有聚赖氨酸处理的载玻片的 24 孔板中。将未经肽处理的细菌用作对照。After incubation, the bacterial suspension was removed, and the treatment method with polylysine-glass slides was repeated. 孵育后,除去细菌悬浮液,并重复用聚赖氨酸-载玻片的处理方法。Then, the polylysine-treated glass slides were fixed with glutaraldehyde (2.5%) at 4 °C for 12 h and dehydrated with a series of graded ethanol solutions for 20 min. The polylysine-treated glass slides were transferred into a mixture (v:v = 1:1) of pure alcohol and tertiary butanol for 15 min, and then placed into pure tertiary butanol for 15 min. 然后,将聚赖氨酸处理的载玻片用戊二醛(2.5%)在4℃下固定12小时,并用一系列梯度乙醇溶液脱水20分钟。将经过聚赖氨酸处理的载玻片转移到纯乙醇和叔丁醇的混合物(v:v = 1:1)中15分钟,然后放入纯叔丁醇中15分钟。Finally, the samples were treated using a critical point dryer with liquid CO2, and then scanned by a S-3400N SEM (Hitachi, Tokyo, Japan) after coating with gold-palladium.最后,使用临界点干燥机用液态 CO2 处理样品,然后在涂上金钯后通过 S-3400N SEM(日本东京日立)进行扫描。
4.12. DNA Binding Assay
The DNA binding assays were determined according to gel retardation experiments described previously [17]. Briefly, 400 ng of genomic DNA (E. coli ATCC25922) was mixed with 0.5 to 256 µM peptide in a binding buffer (50 µg/mL BSA, 1 mM ethylene diamine tetraacetic acid (EDTA), 20 mM KCl, 10 mM Tris-HCl (pH = 8.0), 5% glycerol, and 1 mM dithiothreitol) for 60 min at 37 °C. DNA 结合测定根据先前描述的凝胶阻滞实验确定[17]。简而言之,将 400 ng 基因组 DNA(大肠杆菌 ATCC25922)与 0.5 至 256 µM 肽在结合缓冲液(50 µg/mL BSA、1 mM 乙二胺四乙酸 (EDTA)、20 mM KCl、10 mM Tris- HCl(pH = 8.0)、5% 甘油和 1 mM 二硫苏糖醇)在 37°C 下处理 60 分钟。Subsequently, the samples were examined by 1% agarose gel electrophoresis.随后,通过1%琼脂糖凝胶电泳检查样品。
4.13. LPS Binding Assay
The ability of peptides to bind LPS was detected on a J-810 spectropolarimeter at 25 °C. Briefly, concentration of LPS ranged from 37.5–600 µM, diluted using the double dilution method. The final concentration of peptides in the LPS solution was 150 µM. 在 J-810 分光旋光计上于 25 °C 下检测肽结合 LPS 的能力。简而言之,LPS 的浓度范围为 37.5–600 µM,使用双倍稀释法稀释。 LPS 溶液中肽的最终浓度为 150 µM。The experimental parameters were the same as in Section 4.3.实验参数与第 4.3 节中的相同。
4.14. LAL Assay
Peptide neutralization was evaluated using a quantitative chromogenic limulus amoebocyte lysate (LAL) assay (Xiamen, China) [43]. Briefly, 1 endotoxin unit (EU) of LPS was incubated with two-fold serial dilutions of peptides at 37 °C for 60 min; then, 100 µL of LAL reagent was added to the mixtures. 使用定量显色鲎变形细胞裂解物(LAL)测定法(中国厦门)评估肽中和作用[43]。简而言之,将 1 个内毒素单位 (EU) 的 LPS 与两倍连续稀释的肽在 37°C 下孵育 60 分钟;然后,将 100 µL LAL 试剂添加到混合物中。After additional incubation for 15 min at 37 °C, 100 µL of chromogenic substrate solution was put into the tubes. Subsequently, the mixtures were incubated for another 10 min at 37 °C before adding 500 µL of azo reagent solutions (1, 2, and 3) in turn. 37°C 额外孵育 15 分钟后,将 100 µL 显色底物溶液放入管中。随后,将混合物在 37°C 下再孵育 10 分钟,然后依次添加 500 µL 偶氮试剂溶液(1、2 和 3)。The data were examined by measuring the OD value at 545 nm (Microplate reader, TECAN GENios F129004, Tecan, Salzburg, Austria).通过测量 545 nm 处的 OD 值来检查数据(酶标仪,TECAN GENios F129004,Tecan,Salzburg,Austria)。
4.15. Endotoxin Neutralization Assay
RAW 264.7 cells were put into 24-well plates at a density of 1.0–2.0 × 105 cells/well and stimulated with LPS (100 ng/mL) with (1–64 μM) or without peptide. 将 RAW 264.7 细胞以 1.0–2.0 × 105 个细胞/孔的密度放入 24 孔板中,并用含有 (1–64 μM) 或不含肽的 LPS (100 ng/mL) 刺激。After incubating for 24 h at 37 °C in 5% CO2, the supernatants were collected for analysis of the NO production using Griess reagent and TNF-α levels using ELISA based on the manufacturer’s protocol. 在 37°C、5% CO2 中孵育 24 小时后,收集上清液,使用 Griess 试剂分析 NO 产生,并根据制造商的方案使用 ELISA 分析 TNF-α 水平。The cells treated with LPS only and untreated cells were set as the positive and negative controls, respectively.仅用LPS处理的细胞和未处理的细胞分别设置为阳性对照和阴性对照。
4.16. Statistical Analysis
ANOVA was used to analyze the data through the SPSS 18.0 software (IBM, Chicago, IL, USA). Quantitative data were expressed as the mean ± standard deviation. A p-value < 0.01 was considered to have statistical significance.通过 SPSS 18.0 软件(IBM,芝加哥,伊利诺伊州,美国)使用 ANOVA 分析数据。定量数据表示为平均值±标准差。 p 值 < 0.01 被认为具有统计显着性。
5. Conclusions5。结论
In this study, we designed peptides based on the sequence template XXRXXRRzzRRXXRXX-NH2, where X represents a hydrophobic amino acid (F, I, and L) and zz represents the loop (GG or pG). 在本研究中,我们基于序列模板XXRXXRRzzRRXXRXX-NH2设计了肽,其中X代表疏水性氨基酸(F、I和L),zz代表环(GG或pG)。The designed peptides effectively enhanced cell selectivity because of the introduction of the loop (GG or pG). 由于环(GG 或 pG)的引入,设计的肽有效增强了细胞选择性。Among them, LRpG (X: L, zz: pG) showed the highest average therapeutic index (GMTI = 73.1), with better conditional stability, and it had an additive effect with conventional antibiotics. 其中,LRpG(X:L,zz:pG)平均治疗指数最高(GMTI=73.1),条件稳定性较好,与常规抗生素有相加作用。The antibacterial mechanism study demonstrated that LRpG first destroyed the integrity of the bacterial cell membrane, caused leakage of the cellular content, then entered the cell and bound to the DNA, and ultimately led to bacterial death. 抗菌机制研究表明,LRpG首先破坏细菌细胞膜的完整性,导致细胞内容物渗漏,然后进入细胞并与DNA结合,最终导致细菌死亡。Furthermore, LRpG also had an obvious impact on anti-inflammatory ability. These results indicated that the design and optimization of natural AMPs in this study will be helpful in improving the clinical application of AMPs.此外,LRpG 对抗炎能力也有明显影响。这些结果表明本研究中天然AMPs的设计和优化将有助于提高AMPs的临床应用。
Supplementary Materials
Supplementary materials can be found at https://www.mdpi.com/1422-0067/21/3/1140/s1.补充材料可在 https://www.mdpi.com/1422-0067/21/3/1140/s1 上找到。
Author Contributions作者贡献
Conceptualization, B.J.; funding acquisition, H.M.; supervision, H.M. and X.J.; project administration, H.M. and X.J.; investigation, Z.W., Y.W., Z.P., and L.K.; validation, Y.Z., X.W., and I.M. All authors have read and agreed to the published version of the manuscript.概念化,B.J.;资金收购,H.M.;监督,H.M.和 X.J.;项目管理,H.M.和 X.J.;调查、Z.W.、Y.W.、Z.P. 和 L.K.;验证、Y.Z.、X.W. 和 I.M. 所有作者均已阅读并同意手稿的出版版本。
Funding
This work was supported by the National Natural Science Foundation of China (Grant number: 31572574, 31872519 and 31572555), the National Key Research and Development Plan (Grant number: 2017YFD0501401), the Theme Guidance Project of Development of Science and Technology of Jilin Province (Grant number: 20160204002NY), the Natural Science Foundation of Jilin Province (Grant number: 20180101003JC), the Jilin Programs for Technology Research and Development (Grant number: 20170101025JC and 20170101021JC), and the Jilin Department of Education Programs for Science and Technology Research (Grant number: 201441).该工作得到国家自然科学基金(批准号:31572574、31872519、31572555)、国家重点研发计划(批准号:2017YFD0501401)、吉林省科技发展主题引导项目的资助吉林省自然科学基金项目(批准号:20160204002NY)、吉林省自然科学基金项目(批准号:20180101003JC)、吉林省科技研究发展计划项目(批准号:20170101025JC、20170101021JC)、吉林省教育厅科技攻关项目(授权号:201441)。
Conflicts of Interest利益冲突
The authors declare that no conflict of interest.作者声明不存在利益冲突。
Abbreviations
AMPsAMP | antimicrobial peptides抗菌肽 |
CD | circular dichroism |
MIC | minimal inhibitory concentration最低抑菌浓度 |
TI | theoretical index |
hRBCs | human red blood cells人类红细胞 |
E. coli | Escherichia coli |
FICI | fractional inhibitory concentration index分数抑菌浓度指数 |
SEM | scanning electron microscopy扫描电子显微镜 |
LPS | lipopolysaccharide |
References
1. Van Boeckel, T.P.; Brower, C.; Gilbert, M.; Grenfell, B.T.; Levin, S.A.; Robinson, T.P.; Teillant, A.; Laxminarayan, R. Global trends in antimicrobial use in food animals. Proc. Natl. Acad. Sci. USA 2015, 112, 5649–5654. [Google Scholar] [CrossRef] [Green Version]
2. Wang, J.J.; Dou, X.J.; Song, J.; Lyu, Y.F.; Zhu, X.; Xu, L.; Li, W.Z.; Shan, A.S. Antimicrobial peptides: Promising alternatives in the post feeding antibiotic era. Med. Res. Rev. 2019, 39, 831–859. [Google Scholar] [CrossRef] [PubMed]
3. Tellez, G.A.; Zapata, J.A.; Toro, L.J.; Henao, D.C.; Bedoya, J.P.; Rivera, J.D.; Trujillo, J.Y.; Rivas-Santiago, B.; Hoyos, R.O.; Castano, J.C. Identification, characterization, immunolocalization, and biological activity of lucilin peptide. Acta Trop. 2018, 185, 318–326. [Google Scholar] [CrossRef] [PubMed]
4. Chen, C.X.; Chen, Y.C.; Yang, C.; Zeng, P.; Xu, H.; Pan, F.; Lu, J.R. High selective performance of designed antibacterial and anticancer peptide amphiphiles. ACS Appl. Mater. Interfaces 2015, 7, 17346–17355. [Google Scholar] [CrossRef] [PubMed]
5. Omardien, S.; Brul, S.; Zaat, S.A. Antimicrobial activity of cationic antimicrobial peptides against gram-positives: Current progress made in understanding the mode of action and the response of bacteria. Front. Cell Dev. Biol. 2016, 4, 111. [Google Scholar] [CrossRef]
6. Zhang, Y.H.; Chen, T.W.; Pan, Z.M.; Sun, X.B.; Yin, X.; He, M.; Xiao, S.Y.; Liang, H.J. Theoretical insights into the interactions between star-shaped antimicrobial polypeptides and bacterial membranes. Langmuir. 2018, 34, 13438–13448. [Google Scholar] [CrossRef]
7. Melo, M.N.; Ferre, R.; Castanho, M.A. Antimicrobial peptides: Linking partition, activity and high membrane-bound concentrations. Nat. Rev. Microbiol. 2009, 7, 245–250. [Google Scholar] [CrossRef]
8. Ong, Z.Y.; Wiradharma, N.; Yang, Y.Y. Strategies employed in the design and optimization of synthetic antimicrobial peptide amphiphiles with enhanced therapeutic potentials. Adv. Drug Deliv. Rev. 2014, 78, 28–45. [Google Scholar] [CrossRef]
9. Tripathi, A.K.; Kumari, T.; Tandon, A.; Sayeed, M.; Afshan, T.; Kathuria, M.; Shukla, P.K.; Mitra, K.; Ghosh, J.K. Selective phenylalanine to proline substitution for improved antimicrobial and anticancer activities of peptides designed on phenylalanine heptad repeat. Acta Biomater. 2017, 57, 170–186. [Google Scholar] [CrossRef]
10. Ahmad, A.; Azmi, S.; Srivastava, R.M.; Srivastava, S.; Pandey, B.K.; Saxena, R.; Bajpai, V.K.; Ghosh, J.K. 参考
1.范·博克尔,T.P.;布劳尔,C.;吉尔伯特,M.;格伦菲尔,B.T.;莱文,S.A.;罗宾逊,T.P.;泰兰特,A.; Laxminarayan, R. 食用动物抗菌药物使用的全球趋势。过程。国家。阿卡德。科学。美国 2015 年,112,5649–5654。 [谷歌学术] [交叉引用] [绿色版]
2. 王建军;窦XJ;宋,J。吕,Y.F.;朱X。徐L.;李文正;单,A.S.抗菌肽:后喂养抗生素时代有前途的替代品。医学。资源。 2019 年修订版,39,831–859。 [谷歌学术] [交叉引用] [PubMed]
3. 乔治亚州泰勒斯;萨帕塔,J.A.;托罗,L.J.;河瑙特区;贝多亚,J.P.;里维拉,法学博士;特鲁希略,J.Y.;里瓦斯-圣地亚哥,B.;霍约斯,RO; Castano, J.C. 亮绿素肽的鉴定、表征、免疫定位和生物活性。阿克塔特洛普。 2018, 185, 318–326。 [谷歌学术] [交叉引用] [PubMed]
4. 陈春霞;陈Y.C.;杨,C.;曾,P。徐,H。潘,F。 Lu,J.R.设计的抗菌和抗癌肽两亲物的高选择性性能。 ACS 应用马特。接口 2015, 7, 17346–17355。 [谷歌学术] [交叉引用] [PubMed]
5. 奥马尔丁,S.;布鲁尔,S.; Zaat, S.A. 阳离子抗菌肽对革兰氏阳性菌的抗菌活性:当前在了解细菌的作用方式和反应方面取得的进展。正面。细胞开发。生物。 2016 年 4 月 111 日。[谷歌学术][交叉引用]
6. 张玉华;陈,T.W.;潘,Z.M.;孙X.B.;尹,X。他,M。肖,S.Y.;梁,H.J.星形抗菌多肽与细菌膜之间相互作用的理论见解。朗缪尔。 2018 年,34,13438–13448。 [谷歌学术] [交叉引用]
7.梅洛,明尼苏达州;费雷,R.; Castanho,M.A.抗菌肽:连接分区、活性和高膜结合浓度。纳特。微生物学牧师。 2009 年,7,245–250。 [谷歌学术] [交叉引用]
8. 翁ZY;维拉达玛,N.;杨Y.Y.用于设计和优化具有增强治疗潜力的合成抗菌肽两亲物的策略。副词。药物递送。 2014 年修订版,78,28–45。 [谷歌学术] [交叉引用]
9.特里帕蒂,A.K.;库玛丽,T.;坦登,A.;赛义德,M.;阿夫山,T.;卡图里亚,M.;舒克拉,P.K.;米特拉,K.;戈什,J.K.选择性苯丙氨酸替换为脯氨酸,以改善在苯丙氨酸七肽重复序列上设计的肽的抗菌和抗癌活性。生物材料学报。 2017 年,57,170–186。 [谷歌学术] [交叉引用]
10.艾哈迈德,A.;阿兹米,S.;斯里瓦斯塔瓦,R.M.;斯里瓦斯塔瓦,S.;潘迪,B.K.;萨克塞纳,R.;巴杰派,V.K.;戈什,J.K.Design of nontoxic analogues of cathelicidin-derived bovine antimicrobial peptide BMAP-27: The role of leucine as well as phenylalanine zipper sequences in determining its toxicity. Biochemistry 2009, 48, 10905–10917. [Google Scholar] [CrossRef]
11. Asthana, N.; Yadav, S.P.; Ghosh, J.K. Dissection of antibacterial and toxic activity of melittin: A leucine zipper motif plays a crucial role in determining its hemolytic activity but not antibacterial activity. J. Biol. Chem. 2004, 279, 55042–55050. [Google Scholar] [CrossRef] [PubMed] [Green Version]
12. Kumar, A.; Tripathi, A.K.; Kathuria, M.; Shree, S.; Tripathi, J.K.; Purshottam, R.K.; Ramachandran, R.; Mitra, K.; Ghosh, J.K. 导管素衍生的牛抗菌肽 BMAP-27 的无毒类似物的设计:亮氨酸和苯丙氨酸拉链序列在确定其毒性中的作用。生物化学 2009, 48, 10905–10917。 [谷歌学术] [交叉引用]
11.阿斯塔纳,N.;亚达夫,S.P.;戈什,J.K.蜂毒肽的抗菌和毒性活性剖析:亮氨酸拉链基序在决定其溶血活性而非抗菌活性方面起着至关重要的作用。 J.Biol。化学。 2004, 279, 55042–55050。 [谷歌学术] [交叉引用] [PubMed] [绿色版]
12. 库马尔,A.;特里帕蒂,A.K.;卡图里亚,M.;什里,S.;特里帕蒂,J.K.; Purshottam,R.K.;拉马钱德兰,R.;米特拉,K.;戈什,J.K.Single Amino acid substitutions at specific positions of the heptad repeat sequence of piscidin-1 yielded novel analogs that show low cytotoxicity and in vitro and in vivo antiendotoxin activity. Antimicrob. Agents Chemother. 2016, 60, 3687–3699. [Google Scholar] [CrossRef] [PubMed] [Green Version]
13. Srivastava, R.M.; Srivastava, S.M.; Singh, M.; Bajpai, V.K.; Ghosh, J.K. piscidin-1 七肽重复序列特定位置的单氨基酸取代产生了新型类似物,其显示出低细胞毒性和体外和体内抗内毒素活性。抗菌剂。特工化疗。 2016 年,60,3687–3699。 [谷歌学术] [交叉引用] [PubMed] [绿色版]
13.斯里瓦斯塔瓦,R.M.;斯里瓦斯塔瓦,S.M.;辛格,M.;巴杰派,V.K.;戈什,J.K.Consequences of alteration in leucine zipper sequence of melittin in its neutralization of lipopolysaccharide-induced proinflammatory response in macrophage cells and interaction with lipopolysaccharide. J. Biol. Chem. 2012, 287, 1980–1995. [Google Scholar] [CrossRef] [PubMed] [Green Version]
14. Dong, N.; Ma, Q.Q.; Shan, A.S.; Lv, Y.F.; Hu, W.N.; Gu, Y.; Li, Y.Z. Strand length-dependent antimicrobial activity and membrane-active mechanism of arginine- and valine-rich β-hairpin-like antimicrobial peptides. Antimicrob. Agents Chemother. 2012, 56, 2994–3003. [Google Scholar] [CrossRef] [Green Version]
15. Chou, S.L.; Shao, C.X.; Wang, J.J.; Shan, A.S.; Xu, L.; Dong, N.; Li, Z.Y. Short, multiple-stranded β-hairpin peptides have antimicrobial potency with high selectivity and salt resistance. Acta Biomater. 2016, 30, 78–93. [Google Scholar] [CrossRef]
16. Dong, N.; Chou, S.L.; Li, J.W.; Xue, C.Y.; Li, X.R.; Chen, B.J.; Shan, A.S. Short symmetric-end antimicrobial peptides centered on β-turn amino acids unit improve selectivity and stability. Front. Microbiol. 2018, 27, 2832. [Google Scholar] [CrossRef] [Green Version]
17. Wang, J.J.; Chou, S.L.; Xu, L.; Zhu, X.; Dong, N.; Shan, A.S.; Chen, Z. High specific selectivity and membrane-active mechanism of the synthetic centrosymmetric α-helical peptides with gly-gly pairs. Sci. Rep. 2015, 5, 15963. [Google Scholar] [CrossRef] [Green Version]
18. Chou, S.L.; Wang, J.J.; Shang, L.; Akhtar, M.U.; Wang, Z.H.; Shi, B.M.; Feng, X.J.; Shan, A.S. Short, symmetric-helical peptides have narrow-spectrum activity with low resistance potential and high selectivity. Biomater. Sci. 2019, 7, 2394–2409. [Google Scholar] [CrossRef]
19. Ma, Z.; Yang, J.; Han, J.Z.; Gao, L.; Liu, H.X.; Lu, Z.X.; Zhao, H.Z.; Bie, X.M. Insights into the antimicrobial activity and cytotoxicity of engineered α-helical peptide amphiphiles. J. Med. Chem. 2016, 59, 10946–10962. [Google Scholar] [CrossRef]
20. Shao, C.X.; Tian, H.T.; Wang, T.Y.; Wang, Z.H.; Chou, S.L.; Shan, A.S.; Chen, B.J. Central β-turn increases the cell selectivity of imperfectly amphipathic α-helical peptides. Acta Biomater. 2018, 69, 243–255. [Google Scholar] [CrossRef]
21. Dong, N.; Wang, C.S.; Zhang, T.T.; Zhang, L.; Xue, C.Y.; Feng, X.J.; Bi, C.P.; Shan, A.S. Bioactivity and bactericidal mechanism of histidine-rich β-hairpin peptide against Gram-negative bacteria. Int. J. Mol. Sci. 2019, 20, 3954. [Google Scholar] [CrossRef] [PubMed] [Green Version]
22. Shao, C.X.; Li, W.Z.; Tan, P.; Shan, A.S.; Dou, X.J.; Ma, D.Y.; Liu, C.Y. Symmetrical modification of minimized dermaseptins to extend the spectrum of antimicrobials with endotoxin neutralization potency. Int. J. Mol. Sci. 2019, 20, 1417. [Google Scholar] [CrossRef] [PubMed] [Green Version]
23. Kumar, P.; Kizhakkedathu, J.N.; Straus, S.K. Antimicrobial peptides: Diversity, mechanism of action and strategies to improve the activity and biocompatibility in vivo. Biomolecules 2018, 8, 4. [Google Scholar] [CrossRef] [PubMed] [Green Version]
24. Zelezetsky, I.; Tossi, A. Alpha-helical antimicrobial peptides-using a sequence template to guide structure-activity relationship studies. Biochim. Biophys. Acta 2006, 1758, 1436–1449. [Google Scholar] [CrossRef] [Green Version]
25. Takahashi, D.; Shukla, S.K.; Prakash, O.; Zhang, G. Structural determinants of host defense peptides for antimicrobial activity and target cell selectivity. Biochimie 2010, 92, 1236–1241. [Google Scholar] [CrossRef]
26. Kumar, A.; Mahajan, M.; Awasthi, B.; Tandon, A.; Harioudh, M.K.; Shree, S.; Singh, P.; Shukla, P.K.; Ramachandran, R.; Mitra, K.; et al. 蜂毒肽亮氨酸拉链序列改变对其中和脂多糖诱导的巨噬细胞促炎反应以及与脂多糖相互作用的影响。 J.Biol。化学。 2012, 287, 1980–1995。 [谷歌学术] [交叉引用] [PubMed] [绿色版]
14. 董,N.;马,QQ;单,A.S.;吕,Y.F.;胡文宁;顾,Y。李YZ.富含精氨酸和缬氨酸的β-发夹样抗菌肽的链长度依赖性抗菌活性和膜活性机制。抗菌剂。特工化疗。 2012, 56, 2994–3003。 [谷歌学术] [交叉引用] [绿色版]
15. Chou,S.L.;邵,C.X.;王,J.J.;单,A.S.;徐L.;董,N。李志远短的多链 β-发夹肽具有高选择性和耐盐性的抗菌效力。生物材料学报。 2016 年,30,78–93。 [谷歌学术] [交叉引用]
16. 董,N.;周S.L.;李,J.W.;薛,C.Y.;李X.R.;陈,B.J.;单,A.S.以β-转角氨基酸单元为中心的短对称末端抗菌肽可提高选择性和稳定性。正面。微生物。 2018, 27, 2832. [谷歌学术] [交叉引用] [绿色版]
17. 王俊杰;周S.L.;徐L.;朱X。董,N。单,A.S.; Chen, Z. 具有甘氨酸-甘氨酸对的合成中心对称 α-螺旋肽的高特异性选择性和膜活性机制。科学。 Rep. 2015, 5, 15963. [Google 学术] [CrossRef] [绿色版]
18. Chou,S.L.;王,J.J.;尚,L.;阿赫塔尔,M.U.;王振华;石,B.M.;冯XJ;单,A.S.短的对称螺旋肽具有窄谱活性、低耐药性和高选择性。生物材料。科学。 2019 年,7,2394–2409。 [谷歌学术] [交叉引用]
19.马Z.;杨,J。韩J.Z.;高,L.;刘H.X.;卢,Z.X.;赵慧珍;别,X.M.深入了解工程化 α-螺旋肽两亲物的抗菌活性和细胞毒性。 J. Med。化学。 2016 年,59,10946–10962。 [谷歌学术] [交叉引用]
20. 邵C.X.;田,H.T.;王,T.Y.;王振华;周S.L.;单,A.S.; Chen,B.J. 中央 β 转角增加了不完全两亲性 α 螺旋肽的细胞选择性。生物材料学报。 2018 年,69,243–255。 [谷歌学术] [交叉引用]
21. 董,N.;王,C.S.;张,T.T.;张L。薛,C.Y.;冯XJ;毕,C.P.;单,A.S.富含组氨酸的β-发夹肽对革兰氏阴性菌的生物活性和杀菌机制。国际。 J.莫尔。科学。 2019, 20, 3954. [谷歌学术] [交叉引用] [PubMed] [绿色版]
22. 邵C.X.;李文正;谭,P。单,A.S.;窦XJ;马,D.Y.;刘C.Y.最小化皮抑肽的对称修饰,以扩展具有内毒素中和效力的抗菌谱。国际。 J.莫尔。科学。 2019, 20, 1417. [谷歌学术] [交叉引用] [PubMed] [绿色版]
23. 库马尔,P.;基扎克达图,J.N.;斯特劳斯,S.K.抗菌肽:多样性、作用机制以及提高体内活性和生物相容性的策略。生物分子 2018, 8, 4. [Google 学术] [CrossRef] [PubMed] [绿色版]
24.泽莱泽茨基,I.; Tossi, A. α-螺旋抗菌肽-使用序列模板指导结构-活性关系研究。生物化学。生物物理学。 Acta 2006, 1758, 1436–1449。 [谷歌学术] [交叉引用] [绿色版]
25. 高桥,D.;舒克拉,S.K.;普拉卡什,O.;张,G。宿主防御肽抗菌活性和靶细胞选择性的结构决定因素。生物化学 2010, 92, 1236–1241。 [谷歌学术] [交叉引用]
26. 库马尔,A.;马哈詹,M.;阿瓦斯蒂,B.;坦登,A.; Harioudh,M.K.;什里,S.;辛格,P.;舒克拉,P.K.;拉马钱德兰,R.;米特拉,K.;等人。Piscidin-1-analogs with double L- and D-lysine residues exhibited different conformations in lipopolysaccharide but comparable anti-endotoxin activities. Sci. Rep. 2017, 7, 39925. [Google Scholar] [CrossRef] [Green Version]
27. Lee, J.K.; Park, S.C.; Hahm, K.S.; Park, Y. A helix-PXXP-helix peptide with antibacterial activity without cytotoxicity against MDRPA-infected mice. Biomaterials 2014, 35, 1025–1039. [Google Scholar] [CrossRef]
28. Zhu, X.; Zhang, L.C.; Wang, J.; Ma, Z.; Xu, W.; Li, J.P.; Shan, A.S. Characterization of antimicrobial activity and mechanisms of low amphipathic peptides with different α-helical propensity. Acta Biomater. 2015, 18, 155–167. [Google Scholar] [CrossRef]
29. Wang, J.J.; Chou, S.L.; Yang, Z.Y.; Yang, Y.; Wang, Z.H.; Song, J.; Dou, X.J.; Shan, A.S. Combating drug-resistant fungi with novel imperfectly amphipathic palindromic peptides. J. Med. Chem. 2018, 61, 3889–3907. [Google Scholar] [CrossRef]
30. Park, I.Y.; Cho, J.H.; Kim, K.S.; Kim, Y.B.; Kim, M.S.; Kim, S.C. Helix stability confers salt resistance upon helical antimicrobial peptides. J. Biol. Chem. 2004, 279, 13896–13901. [Google Scholar] [CrossRef] [Green Version]
31. Jin, Y.; Hammer, J.; Pate, M.; Zhang, Y.; Zhu, F.; Zmuda, E.; Blazy, J. Antimicrobial activities and structures of two linear cationic peptide families with various amphipathic β-sheet and α-helical potentials. Antimicrob. Agents Chemother. 2005, 49, 4957–4964. [Google Scholar] [CrossRef] [PubMed] [Green Version]
32. Arouri, A.; Dathe, M.; Blume, A. The helical propensity of KLA amphipathic peptides enhances their binding to gel-state lipid membranes. Biophys. Chem. 2013, 180, 10–21. [Google Scholar] [CrossRef] [PubMed]
33. Ma, Q.Q.; Dong, N.; Shan, A.S.; Lv, Y.F.; Li, Y.Z.; Chen, Z.H.; Cheng, B.J.; Li, Z.Y. Biochemical property and membrane-peptide interactions of de novo antimicrobial peptides designed by helix-forming units. Amino Acids 2012, 43, 2527–2536. [Google Scholar] [CrossRef]
34. Khara, J.S.; Wang, Y.; Ke, X.Y.; Liu, S.; Newton, S.M.; Langford, P.R.; Yang, Y.Y.; Ee, P.L. Anti-mycobacterial activities of synthetic cationic α-helical peptides and their synergism with rifampicin. Biomaterials 2014, 35, 2032–2038. [Google Scholar] [CrossRef]
35. Liu, Y.F.; Xia, X.; Xu, L.; Wang, Y.Z. Design of hybrid β-hairpin peptides with enhanced cell specificity and potent anti-inflammatory activity. Biomaterials 2013, 34, 237–250. [Google Scholar] [CrossRef] [PubMed]
36. Hancock, R.E.; Haney, E.F.; Gill, E.E. The immunology of host defence peptides: Beyond antimicrobial activity. Nat. Rev. Immunol. 2016, 16, 321–334. [Google Scholar] [CrossRef]
37. Cohen, J.; Vincent, J.L.; Adhikari, N.K.; Machado, F.R.; Angus, D.C.; Calandra, T.; Jaton, K.; Giulieri, S.; Delaloye, J.; Opal, S.; et al. Sepsis: A roadmap for future research. Lancet Infect. Dis. 2015, 15, 581–614. [Google Scholar] [CrossRef]
38. Zong, X.; Song, D.; Wang, T.; Xia, X.; Hu, W.; Han, F.; Wang, Y. LFP-20, a porcine lactoferrin peptide, ameliorates LPS-induced inflammation via the MyD88/NF-κB and MyD88/MAPK signaling pathways. Dev. Comp. Immunol. 2015, 52, 123–131. [Google Scholar] [CrossRef]
39. Khara, J.S.; Obuobi, S.; Wang, Y.; Hamilton, M.S.; Robertson, B.D.; Newton, S.M.; Yang, Y.Y.; Langford, P.R.; Ee, P.L.R. Disruption of drug-resistant biofilms using, de novo, designed short α-helical antimicrobial peptides with idealized facial amphiphilicity. Acta Biomater. 2017, 57, 103–114. [Google Scholar] [CrossRef]
40. Wang, J.J.; Song, J.; Yang, Z.Y.; He, S.Q.; Yang, Y.; Feng, X.J.; Dou, X.J.; Shan, A.S. Antimicrobial peptides with high proteolytic resistance for combating gram-negative bacteria. J. Med. Chem. 2019, 62, 2286–2304. [Google Scholar] [CrossRef]
41. Dong, N.; Zhu, X.; Chou, S.; Shan, A.S.; Li, W.Z.; Jiang, J.G. Antimicrobial potency and selectivity of simplified symmetricend peptides. Biomaterials 2014, 27, 8028–8039. [Google Scholar] [CrossRef] [PubMed]
42. Lv, X.T.; Ma, Q.Q.; Zhu, D.D.; Shao, C.X.; Lv, Y.F.; Shan, A.S. The C-terminal sequences of porcine thrombin are active as antimicrobial peptides. Chem. Biol. Drug Des. 2016, 88, 905–914. [Google Scholar] [CrossRef] [PubMed]
43. Sun, Y.; Dong, W.B.; Sun, L.; Ma, L.J.; Shang, D.J. Insights into the membrane interaction mechanism and antibacterial properties of chensinin-1b. Biomaterials 2015, 37, 299–311. [Google Scholar] [CrossRef] [PubMed]具有双L-和D-赖氨酸残基的Piscidin-1-类似物在脂多糖中表现出不同的构象,但具有相当的抗内毒素活性。科学。 Rep. 2017, 7, 39925。 [Google 学术] [CrossRef] [绿色版]
27.李,J.K.;南卡罗来纳州帕克;哈姆,K.S.; Park, Y. 一种螺旋-PXXP-螺旋肽,具有抗菌活性,对 MDRPA 感染的小鼠没有细胞毒性。生物材料 2014, 35, 1025–1039。 [谷歌学术] [交叉引用]
28. 朱X.;张,L.C.;王,J。马,Z。徐,W。李,J.P.;单,A.S.具有不同α-螺旋倾向的低两亲性肽的抗菌活性和机制的表征。生物材料学报。 2015 年,18,155–167。 [谷歌学术] [交叉引用]
29.王JJ;周S.L.;杨,Z.Y.;杨,Y。王振华;宋,J。窦XJ;单,A.S.用新型不完全两亲性回文肽对抗耐药真菌。 J. Med。化学。 2018, 61, 3889–3907。 [谷歌学术] [交叉引用]
30.帕克,I.Y.;曹,J.H.;金,K.S.;金,Y.B.;金,硕士; Kim, S.C. 螺旋稳定性赋予螺旋抗菌肽耐盐性。 J.Biol。化学。 2004, 279, 13896–13901。 [谷歌学术] [交叉引用] [绿色版]
31.金,Y.;哈默,J.;佩特,M.;张,Y。朱F.;兹穆达,E.; Blazy, J. 具有各种两亲性 β-折叠和 α-螺旋电位的两个线性阳离子肽家族的抗菌活性和结构。抗菌剂。特工化疗。 2005 年,49,4957–4964。 [谷歌学术] [交叉引用] [PubMed] [绿色版]
32.阿鲁里,A.;达瑟,M.; Blume, A. KLA 两亲肽的螺旋倾向增强了它们与凝胶态脂质膜的结合。生物物理学。化学。 2013 年,180,10-21。 [谷歌学术] [交叉引用] [PubMed]
33.马,Q.Q.;董,N。单,A.S.;吕,Y.F.;李,Y.Z.;陈振华;程,B.J.;李志远由螺旋形成单元设计的从头设计的抗菌肽的生化特性和膜-肽相互作用。氨基酸 2012, 43, 2527–2536。 [谷歌学术] [交叉引用]
34.卡拉,J.S.;王,Y。柯,X.Y.;刘,S。牛顿,S.M.;兰福德,P.R.;杨,Y.Y.; Ee,P.L.合成阳离子α-螺旋肽的抗分枝杆菌活性及其与利福平的协同作用。生物材料 2014, 35, 2032–2038。 [谷歌学术] [交叉引用]
35.刘YF;夏,X。徐L.;王YZ具有增强细胞特异性和有效抗炎活性的杂合 β-发夹肽的设计。生物材料 2013, 34, 237–250。 [谷歌学术] [交叉引用] [PubMed]
36.汉考克,R.E.;哈尼,E.F.; Gill,E.E.宿主防御肽的免疫学:超越抗菌活性。纳特。免疫学牧师。 2016 年,16,321–334。 [谷歌学术] [交叉引用]
37. 科恩,J.;文森特,J.L.;阿迪卡里,N.K.;马查多,F.R.;安格斯特区;卡兰德拉,T.;贾顿,K.;朱列里,S.;德拉洛耶,J.;蛋白石,S.;等人。脓毒症:未来研究的路线图。柳叶刀感染。迪斯。 2015 年,15,581–614。 [谷歌学术] [交叉引用]
38.宗X.;宋,D.;王,T。夏,X。胡,W。汉,F。 Wang, Y. LFP-20 是一种猪乳铁蛋白肽,通过 MyD88/NF-κB 和 MyD88/MAPK 信号通路改善 LPS 诱导的炎症。开发。比较。免疫学。 2015 年,52,123–131。 [谷歌学术] [交叉引用]
39.卡拉,J.S.;奥布比,S.;王,Y。汉密尔顿,硕士;罗伯逊,B.D.;牛顿,S.M.;杨,Y.Y.;兰福德,P.R.; Ee,P.L.R。使用从头设计的具有理想面部两亲性的短 α-螺旋抗菌肽破坏耐药生物膜。生物材料学报。 2017 年,57,103–114。 [谷歌学术] [交叉引用]
40.王JJ;宋,J。杨,Z.Y.;他,S.Q.;杨,Y。冯XJ;窦XJ;单,A.S.具有高蛋白水解抗性的抗菌肽,可对抗革兰氏阴性菌。 J. Med。化学。 2019, 62, 2286–2304。 [谷歌学术] [交叉引用]
41. 董,N.;朱X。周,S。单,A.S.;李文正;江J.G.简化对称末端肽的抗菌效力和选择性。生物材料 2014, 27, 8028–8039。 [谷歌学术] [交叉引用] [PubMed]
42. Lv,X.T.;马,QQ;朱,D.D.;邵,C.X.;吕,Y.F.;单,A.S.猪凝血酶的 C 末端序列具有抗菌肽的活性。化学。生物。药物设计。 2016 年,88,905–914。 [谷歌学术] [交叉引用] [PubMed]
43.孙Y.;董W.B.;孙,L.;马,L.J.;尚,D.J.深入了解 chensinin-1b 的膜相互作用机制和抗菌特性。生物材料 2015, 37, 299–311。 [谷歌学术] [交叉引用] [PubMed]
© 2020 by the authors. Licensee MDPI, Basel, Switzerland. This article is an open access article distributed under the terms and conditions of the Creative Commons Attribution (CC BY) license (http://creativecommons.org/licenses/by/4.0/).© 2020 作者版权所有。被许可方 MDPI,瑞士巴塞尔。本文是根据知识共享署名 (CC BY) 许可证 (http://creativecommons.org/licenses/by/4.0/) 的条款和条件分发的开放获取文章。
- Author:Tongtou
- URL:https://www.monet267.top//article/8fcb32c1-229b-43cf-a1a0-232958891a2c
- Copyright:All articles in this blog, except for special statements, adopt BY-NC-SA agreement. Please indicate the source!